Biofluorescent particle counter-based real-time feedback and control of processing conditions
Posted: 3 September 2019 | Aditya Prasad (AstraZeneca), Allison Scott (Azbil North America R&D – BioVigilant), Deward Manzer (MicronView), Jeffrey Weber (Pfizer Inc), Jih-Peng Chen (Biogen), Lisa Lawson (Particle Measuring Systems), Mike Dingle (TSI), Peter Noverini (Baxter Healthcare Corporation), Philip Villari (Merck and Co), Scott Hooper (MSD) | No comments yet
Biofluorescent particle detectors offer a fundamentally different way of enumerating microbes present in an environment, as compared to traditional growth-based methods. Here, the Process and Environmental Monitoring Methods (PEMM) group shares information about the technology and applications for real-time biofluorescent particle detection methods and how they help to address major industry concerns.
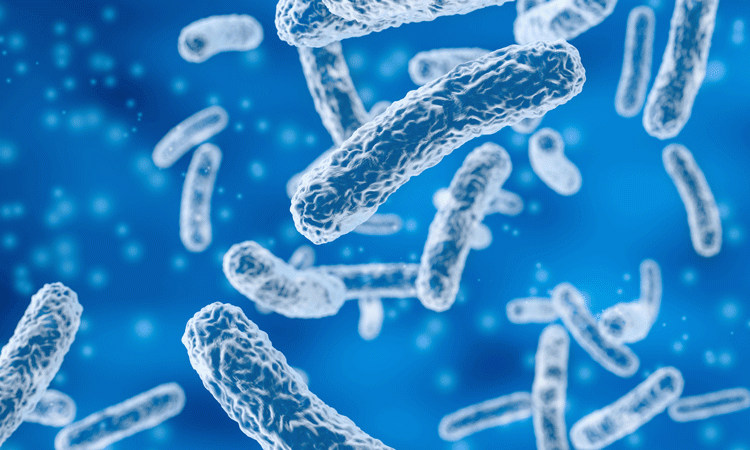
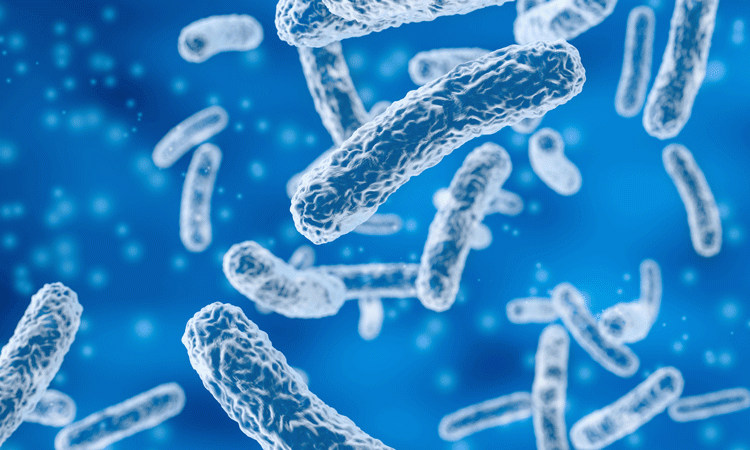
BIOLOGIC FLUORESCENCE (also referred to as biological autofluorescence) is based on the laser excitation and emission of biological compounds such as nicotinamide adenine dinucleotide (NADH), riboflavin, tryptophan and picolinic acid. One or more of these compounds is found in all microorganisms.1
Biofluorescent particle (BFP) detection systems are a form of enhanced particle counter, capable of the continuous and real-time detection of inert particles and microorganisms in both air and pharmaceutical-grade waters. These systems utilise the detection of scattered laser light for particle enumeration and fluorescence detection for the classification of detected particles as either BFP (ie, biologic) or inert (ie, non-biologic) (Figure 1). As particles are sampled by the BFP counting system they are exposed to laser light, commonly with a 405nm wavelength. Inert particles scatter this light as they travel through the laser beam. BFPs both scatter the laser light and emit fluorescence. This emitted fluorescence is the result of laser light absorption by fluorescent molecules within the particle followed by emission at a longer wavelength. This process is known as laser-induced fluorescence (LIF). LIF can be used to excite fluorescent molecules present in all microorganisms, including NADH, dipicolinic acid and riboflavin, when 405nm excitation is used. The BFP detection systems currently available are designed to have the sensitivity and specificity to detect light emission from such fluorescent molecules found in microorganisms.
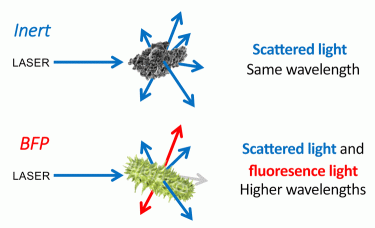
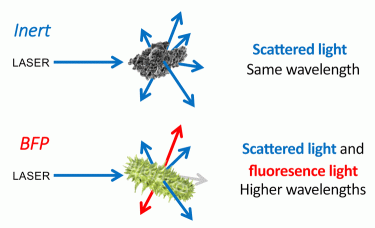
Figure 1: BFP detection systems utilise the detection of scattered laser light for particle enumeration and fluorescence detection for the classification of detected particles as either BFP (ie, biologic) or inert (ie, non-biologic).
BFP detectors offer a fundamentally different way of enumerating microbes present in an environment, as compared to traditional growth‑based methods. BFP detectors use information about size and the natural fluorescence of a particle to indicate a microbe is present. This unit of measure is often termed an autofluorescent unit (AFU) due to its dependence on natural fluorescence. Detection based on autofluorescence removes the need for sample preparation, such as staining in the case of LIF-based flow cytometry, and better enables the detection of viable but nonculturable microorganisms since it is not dependent upon growth.2 Although BFP detection systems are designed to minimise the classification of non‑biologic fluorescent particles as biologic, like some plastics, it is possible that some non‑biologic fluorescent particles will be classified as a BFP and reported as an AFU. By comparison, the colony forming unit (CFU) is based upon growth and used to estimate the number of viable and culturable bacteria or fungal cells in a sample. However, many microorganisms are viable but non-culturable on traditional media under standard incubation conditions, therefore methods based on culturability can have significant limitations regarding the number of organisms detected. Owing to these differences in detection, an AFU is not equivalent to a CFU, but rather the methods can be considered complementary.
Through the continuous monitoring of total and BFP counts, and autofluorescence-based detection, BFP detection systems offer an often higher sensitivity than traditional methods and real‑time insight into microbes present in air or water. This continuous and data-rich source of microbial information can be used to increase process understanding and control and has resulted in BFP detection systems being used in a number of real‑time air and water monitoring applications.
Practical implementation
BFP detectors offer a fundamentally different way of enumerating microbes present in an environment, as compared to traditional growthbased methods”
The pharmaceutical industry quickly identified the potential value of BFP systems’ ability to monitor changes in classified environments and intermittent events, and in continuous monitoring.3-5 There are numerous opinions about the roles and implementation of BFP technology in manufacturing, quality oversight and regulatory acceptance. Implementation has required a shift toward collaborative development of best practices to accelerate the adoption of the systems. Open sharing of datasets and analysis can create an alignment of expectations and appropriate responses from internal quality groups and external regulatory agencies. The BFP instruments have enabled a paradigm shift for continuous monitoring with continuous trending data and feedback enabling real-time operational actions to address changes detected in the environment. This contrasts with the traditional, intermittent culture-based results that are an assessment of whether bioburden CFUs are within acceptable limits at a single point in time.
Portable BFP analysers are process analytical technologies (PAT) that can be brought to the shop floor for air and water monitoring. The benefit of portable, continuous analysers is that they enable real-time process monitoring of aseptic manufacturing and differentiate BFPs (both air and water) from traditional laboratory techniques.6,7
Case studies
Several companies have successfully used portable BFP analysers in non-GMP monitoring applications. While a few of these uses have been published, the authors are aware of many additional unpublished non-GMP applications.
Comparison of the performance of an air-based BFP analyser and both Andersen air samplers and an all-gas impingement method coupled with reagent-based fluorescent cell counting in controlled chambers and classified and unclassified areas showed that the air-based BFP analyser had recovery rates equal to or better than the other two methods.8
In 2014, Sandle et al. described the use of a BFP air analyser to provide real-time assurance and found that a controlled environment maintained suitable conditions through standard operation, shutdown and maintenance; cleaning and disinfection; before return to normal use.9 Through these studies they showed the utility of BFP systems for establishing a baseline while in normal operation against which post-shutdown conditions can be measured to provide assurance that the area or utility is ready to be successfully requalified as suitable for use. They also point out that BFP analysers could be used as risk mitigation tools to provide assurance that the area or utility could be released on risk based on initial data (eg, particulate counts), but before the completion of incubation times for viable air plates.
BFP detection systems offer an often higher sensitivity than traditional methods and real-time insight into microbes present in air or water”
Implementation of a water-based BFP analyser in a new high-purity water system was described by Lipko, Termine and Walter.10 The analyser was placed on the HPW distribution system to complement the on-line total organic carbon (TOC) and conductivity measurements. The authors claimed the BFP analyser allowed a 20 percent reduction in the number of grab samples that needed to be taken and assayed.
José-Miguel Montenegro-Alvarado recently described how a BFP air analyser system was used following a hurricane-induced long-duration facility outage to establish contamination focus areas for special remediation prior to formal re-establishment of cleanroom conditions.11 These activities included a grid-based survey of EM conditions, survey of opening and closing doors between rooms and into cabinets to map bioburden flux, scanning of specific areas of concern and ceiling fixtures and a re-scanning of areas after remediation and cleaning prior to formal sampling for re-establishing room classification.
In a second publication, Montenegro- Alvarado et al. described, also in the context of restoring facilities to operational readiness following an extended outage, the use of BFP analysers to provide assurance prior to formal requalification.12 These activities included scanning HEPA filters for particulate leakage and replacement prior to formal requalification activities, checking cleaned and disinfected rooms for formal requalification readiness and the use of a BFP water analyser for the assessment of bioburden in purified water systems.
Current challenges
For high-stringency uses like grade A air or WFI, the need to identify and control single-detection events becomes critical. To distinguish true viable microbe detection from false positives, sample capture or not‑yet-implemented alternative identification methods can be used. It is, however, currently impractical to capture and identify single particle events in a manufacturing facility. The development of sample capture systems that provide reasonable controls to identify potential viable microorganisms will aid appropriate responses. A recent publication provides user requirement specifications for water‑based BFP analysers and may be useful for ensuring that baseline expectations are met.13
BFP instruments are validated using the same principles as traditional particle counters to ensure reliable particle counts. The complementarity, but not direct correlation, of AFU and CFU presents challenges in explaining the difference between the growth-based CFU measurement to the more holistic AFU measurement system. Appropriate alert and action levels should be developed by industry based on the baseline AFU counts obtained in their environment, the stringency of the intended application and the potential and risk of a single particle event during a manufacturing operation.
Where do we go from here? The BFP‑illuminated future of manufacturing
The long history of the BFP methodology and these published examples provides evidence and assurance that BFP technologies have utility in non-good manufacturing practice (GMP) settings and currently (with proper caution) also in GMP settings. Analysers have been deployed to monitor air and water under a wide variety of conditions in both non-GMP and GMP settings. Some of these uses, such as remediation of a facility following a catastrophic event such as a major hurricane, are both clever and wise as the real-time data obtained from BFP analysers provides immediate feedback on conditions. The increase in recent publications on the use of BFP analysers has been significant and this group looks forward to seeing additional publications covering the use of BFP analysers as they will increase the collective body of knowledge, lead to even greater acceptance of the technology and reveal novel ways that the technology can be leveraged to monitor manufacturing facilities and process stream conditions.
About the authors
The authors comprise a collaborative group formed to improve the acceptability and use of real-time biofluorescent particle detection process and environmental monitoring methods (PEMM) for air and water in the pharmaceutical industry. With that aim, the team has sought to share knowledge on the technology behind and applications for real-time biofluorescent particle detection methods and their benefits to address major industry concerns such as process understanding, risk management, productivity and safety.
Fred Ayers, Research Scientist, Eli Lilly.
Jih-Peng Chen, Quality Control Associate III, Biogen.
Mike Dingle, Field Application Specialist, TSI.
Scott Hooper, Associate Director, MSD.
Lisa Lawson, Global Pharma GMP Adviser, Particle Measuring Systems.
Deward Manzer, President and CEO, MicronView.
Peter Noverini, Research Associate III, Baxter Healthcare Corporation.
Aditya Prasad, QC Lead, AstraZeneca.
Allison Scott, Senior Principal Scientist, Azbil North America R&D – BioVigilant.
Philip Villari, Associate Principal Scientist, Merck and Co., Inc.
Jeffrey Weber, Senior PAT Project Manager, Pfizer Inc.
References
- Ammor MS. Recent advances in the use of intrinsic fluorescence for bacterial identification and characterization. J Fluor. 2007 Oct;17(5):455-59.
- Irie K, Scott A, Haseqawa N. Investigation of the detection ability of an intrinsic fluorescence-based bioaerosol detection system for heat-stressed bacteria. PDA J Pharm Sci Technol. 2014 Sep/Oct;68(5):478-93.
- Miller MJ, Lindsay H, Valverde-Ventura R, O’Connor MJ. Evaluation of the BioVigilant IMD-A, a novel optical spectroscopy technology for the continuous and real-time environmental monitoring of viable and nonviable particles. Part I: Review of the technology and comparative studies with conventional methods. PDA J Pharm Sci Technol. 2009 May/Jun;63(3):245-258.
- Cundell A, Gordon O, Haycocks N, Johnston J, Luebke M, Lewis N, et al. Novel concept for online water bioburden analysis: key considerations, applications, and business benefits for microbiological risk reduction. Amer Pharm Rev. 2013;16(3):26-31.
- Moldenhauer J. Environmental monitoring, a comprehensive handbook. 2nd ed. River Grove: DHI Publishing LLC; 2005. 459p.
- Sandle T. Real-time biological particle counting in environmental monitoring. Eur. Pharm. Rev. [Internet]. 2015 Apr [cited 2019 Apr 21];2. Available from: https://www.europeanpharmaceuticalreview.com/article/30900/real-time-biological-particle-counting-in-environmental-monitoring/
- Anders HJ, Ayers F, Fitch B, Forng RY, Hooper S, Luebke M, et al. Practical application of online water bioburden analyzers in pharmaceutical manufacturing. Pharm Online [Internet]. 2017 Jul [cited 2019 Apr 21]. Available from: https://www.pharmaceuticalonline.com/doc/practical-application-of-online-water-bioburden-analyzers-in-pharmaceutical-manufacturing-0001
- Bhupathiraju VK, Jiang JP, Bolotin C, Varnau B, Nelson JR. Evaluation of an instantaneous microbial detection system in controlled and cleanroom environments. BioPharm Int [Internet]. 2007 Sep [cited 2019 Apr 21]. 20(9):35-46. Available from: http://www.biopharminternational.com/evaluation-instantaneous-microbial-detection-system-controlled-and-cleanroom-environments
- Sandle T, Leavy C, Jindal H, Rhodes R. Application of rapid microbiological methods for the risk assessment of controlled biopharmaceutical environments. J Appl Microbiol. 2014 Jun;116(6):1495-505.
- Lipko B, Termine B, Walter S. 2017. Case study: retrofitting two new high-purity water systems [Internet]. Pharm Tech; 2017 [cited 2019 Apr 22]. ps18-s26. Available from: http://www.pharmtech.com/case-study-retrofitting-two-new-high-purity-water-systems-0
- Montenegro-Alvarado JM. Leveraging rapid microbiological methodology in forensic evaluation to identify elusive root cause. Amer Pharm Rev [Internet]. 2018 Sep [cited 2019 Apr 21]. Available from: https://www.americanpharmaceuticalreview.com/Featured-Articles/353500-Leveraging-Rapid-Microbiological-Methodology-in-Forensic-Evaluation-to-Identify-Elusive-Root-Cause/
- Montenegro-Alvarado JM, Salvas J, Weber J, Mejías S, Arroyo R. Pfizer case study: rapid microbial methods for manufacturing recovery after Hurricane María. Pharm Online [Internet]. 2018 July [cited 2019 Apr 21]. Available from: https://www.pharmaceuticalonline.com/doc/pfizer-case-study-rapid-microbial-methods-for-manufacturing-recovery-after-hurricane-mar-a-0001
- Online Water Bioburden Analyzer Workgroup. A better approach to pharmaceutical water testing – user requirements for an online water bioburden analyzer. Pharm Online [Internet]. 2018 Nov [cited 2019 Apr 21]. Available from: https://www.pharmaceuticalonline.com/doc/a-better-approach-to-pharmaceutical-water-testing-user-requirements-for-an-online-water-bioburden-analyzer-0001