Hitting the target – refining anticancer nanomedicine development
Posted: 3 September 2019 | Dr Sarwar Beg (Hamdard University), Mahfoozur Rahman (Sam Higginbottom University of Agriculture) | No comments yet
Mahfoozur Rahman and Sarwar Beg explain why we need to change tack from traditional formulation-driven development to a target-based approach in order to increase success with anticancer nanomedicine in the clinic.
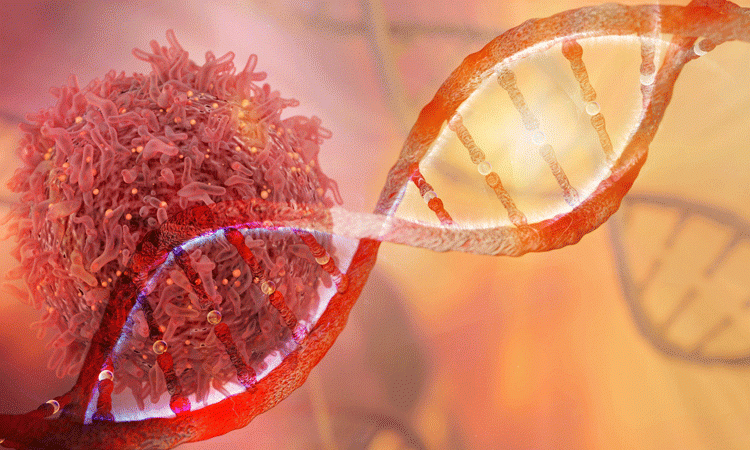
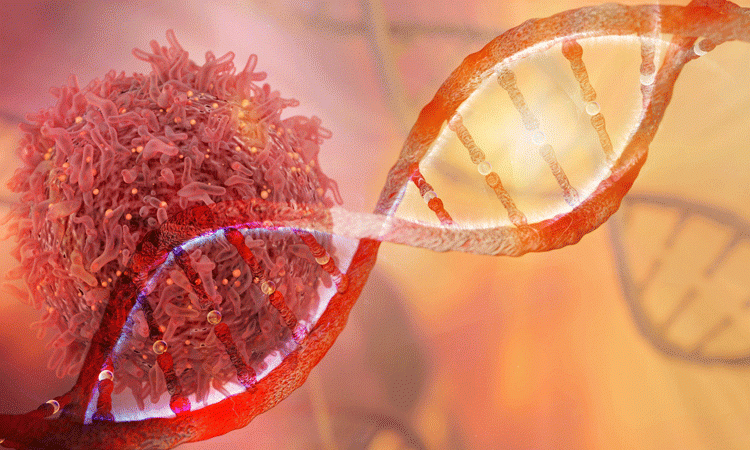
THE SUCCESSFUL translation of anticancer nanomedicine from its genesis to therapeutic outcome in the clinic is challenging. However, despite significant advancements in the realm of drug delivery, we still need better understanding of oncology drug development and target-driven design for improved patient outcomes. The success of anticancer nanomedicines as targeted therapies has been significantly improved by applying the ‘5R strategy’ – right target/efficacy, right tissue/exposure, right safety, right patient and right commercial potential. Establishing these Rs requires the collection of data packages, which is achieved by garnering the following information:
- in-depth knowledge of the heterogeneity of cancers and biological factors that influence the behaviour of nanomedicine towards tumours
- switch onto disease-driven development from a formulation-driven approach
- searching for the most appropriate animal model and testing protocols
- pre-selection of those patients who will suit nanomedicine therapies.
These are the steps that must be undertaken to overcome the challenges encountered in nanomedicine development and translation to make the best therapies for cancer patients in a cost-effective way.
Moving from formulation- to disease-driven design
Pre-clinical experimentation is vital in the setup and optimisation of new nanomedicines and aims to identify weaknesses in the development of nanomedicine. It also ensures therapeutic capability and highlights any potential clinical risk in the formulations.1 Previously, the development of anticancer nanomedicines used the standard formulation-driven approach, which includes evaluating formulations using in vitro cytotoxicity, in vivo biopharmaceutical studies and antitumour experiments in xenograft models. However, this framework has not generated the necessary data to enable the successful translation of nanomedicines to the clinic.1 Before the financial investment stage, we need to change current nanomedicine development strategies and this means focusing on disease-driven design, generating pre-clinical data to inform clinically relevant therapeutic endpoints, which can be used to treat the right patients.1
Adopting a structured approach
Pharma researchers should test a focused hypothesis and proceed only with those agents that are most likely to progress with the least cost”
No nanomedicine has achieved all the necessary properties to achieve the right efficacy for different tumours. Therefore, we must adopt a disease-driven development strategy and transition away from formulation-driven approaches. From the beginning of the project, assess the clinical line of sight and ensure an understanding of the specific challenges of the cancer, such as abnormal organ toxicity and abnormal pharmacokinetic profile. Designing a nanomedicine on a structural basis for a specific tumour leads to a higher probability of success compared to the sole development of formulations alone.2 So, it is important to establish a rationale for development of a successful nanomedicine.
It is important to understand the disease-driven approach, which requires understanding the kinetics of a nanomedicine in a cancer patient. The heterogeneity of different tumours is certainly a hindrance to nanomedicine, but this may change by using surface-anchored/specifically-designed nanomedicine.2,3 This approach led to understanding the importance of finding the ‘right nanomedicine for the right patient’.
Patient-focused design
Identifying the off-target effects of nanomedicines is important in evaluating the efficacy of nanomedicines. These factors include distribution into tissues and accumulations in the reticuloendothelial system.3 Furthermore, the biodistribution of drugs in off-target organs can be minimised by controlled drug release formulation and the use of relative clearance. An example of an Aurora-B kinase inhibitor is AstraZeneca’s AZD2811, which is delivered using the BIND Therapeutics Accurin™ polymeric nanoparticle. The drug release-controlled rate of the nanomedicine minimises bone marrow toxicity and improves drug efficacy. Moreover, the same efficacy can be seen in a rat xenograft model at different controlled drug release rates with different levels of bone marrow toxicity.4,5
Stronger platform of evidence
Drug accumulation in the tumour by enhanced permeability and retention (EPR) effects has been reported by using nanomedicine, as revealed in various literatures; while in other types of cancer, the accumulation of nanocarriers has not been at a satisfactory level. Furthermore, alterations made in the pharmacokinetics of anticancer drugs results in better therapeutics.4,5 Therefore, for determination of nanomedicine efficacy, four parameters need to be studied: tumour accumulation, intratumoural distribution, tumour retention of the system and peripheral pharmacokinetics of the nanoformulation.4,5 In any tumour, these four features may play an independent role in contributing to potential drug efficacy; while the prominent feature will influence the choice of better dosage forms that offer optimum pharmacokinetics.
Employment of more clinically relevant models
Patient-derived tumour explants (PDX) are genetically modified mouse models with more features,6,7 however, they are difficult to maintain due to their slow growth in their given environment. GEMMS is a different model, which unfortunately experiences a similar issue to the PDX model.6,7 However, there are several other models available through contract research organisations (CROs) and academic institutions. There have been various studies reported on the use of such models for nanomedicines, which give new perspectives. All these above factors are depicted in Figure 1.
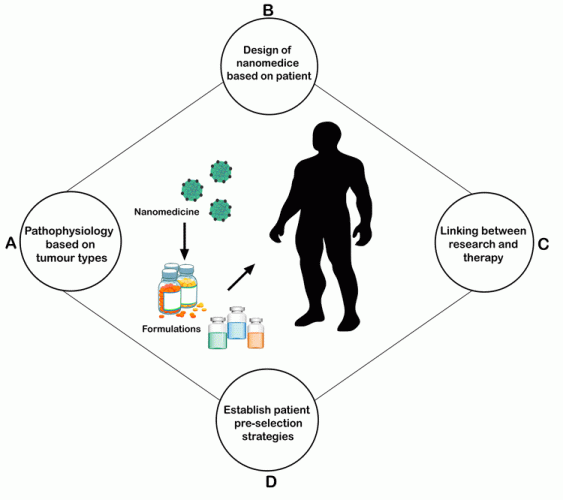
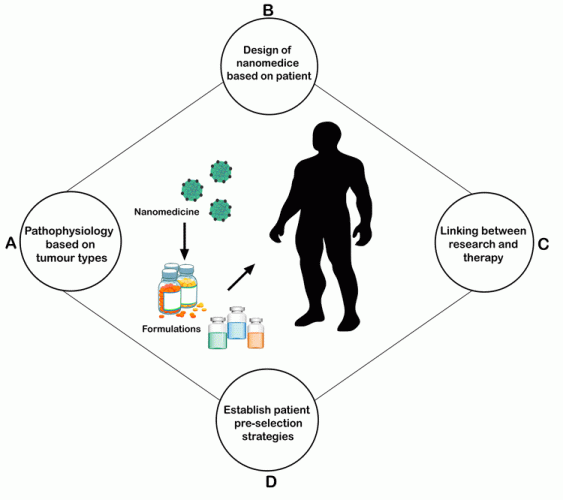
Figure 1: Steps involved in the successful development of nanomedicines.
Pharma perspectives
Multiple challenging questions remain in oncology projects and the following are usually asked: why is the pre-clinical testing of anticancer nanomedicines so poor at predicting their clinical potential? Why is there such a marked difference between animal models of cancer and human patients? What parameters must be considered pre-clinically to improve success clinically?
The probability of success of an anticancer drug starting from pre-clinical proof-of-principle to commercial launch is only in the range of six percent”
The teams involved in cancer research need to define the types of tumour they are targetting, their stage, dose and dosing schedule, with current use of drugs or other agents given in combinations. Furthermore, they should also investigate the risks that could potentially hinder clinical success.8-10 If we can answer the above questions and address targeting issues, we can enhance the translation of oncology therapeutics and save large amounts of unnecessary investment. To make a project attractive, pharma researchers should test a focused hypothesis and proceed only with those agents that are most likely to progress with the least cost. Any anticancer drug molecule project should be based on the 5R strategy discussed earlier.10 This general approach has been shown to improve clinical translation of anticancer nanomedicine.
Cost of success and failure
The probability of success of an anticancer drug starting from pre-clinical proof-of-principle to commercial launch is only in the range of six percent. There is continuing demand for innovative, patient-personalised therapeutics to improve clinical outcomes. Nanomedicines offer tremendous potential to achieve this goal, but industry must make the right decisions when investing in nanomedicines and drugs such as peptides and DNA/RNA-based agents.10 Due to a lack of understanding and establishment between critical quality attributes and efficacy, these formulations are challenging for anticancer drugs. Furthermore, the regulatory landscape and perceptions are constantly evolving; manufacturing and scale up are technically challenging; key analytical methods require further advancement; and the cost of goods, such as tablets and standard parenteral instruments, is high. A key consideration when adopting a nanomedicine is their cost versus the cheaper (often generic) therapy; so if such approaches can be delivered cost effectively with the right commercial potential, the advantages should be realised.10
Collaborations needed for nanomedicine success
The development of anticancer nanomedicine therapeutics is a multidisciplinary endeavour. It requires in-depth integration of interdisciplinary sciences with nanotechnology in medicine. Moreover, the complexity of nanomedicine development is varied and requires different expertise. It therefore requires collaboration between large pharma, small industries, cancer research hospitals and academia to carry out adequate pre-clinical development, utilising the unique skills of each collaborator.10
About the authors
References
- Chauhan VP, Jain RK. Strategies for advancing cancer nanomedicine. Mater. 2013; 12(11): 958-962.
- Kanapathipillai M, Brock A, Ingber DE. Nanoparticle targeting of anti-cancer drugs that alter intracellular signalling or influence the tumour microenvironment. Adv Drug Deliv Rev. 2014; 79-80: 107-118.
- Chauhan VP, Stylianopoulos T, Martin JD, et al. Normalization of tumour blood vessels improves the delivery of nanomedicines in a size-dependent manner. Nat Nanotechnol. 2012; 7(6):383-388.
- Kirtane AR, Siegel RA, Panyam J Pharmacokinetic model for quantifying the effect of vascular permeability on the choice of drug carrier: a framework for personalized nanomedicine. J Pharm Sci. 2015; 104(3): 1174-1186.
- Ashton S, Taylor P, Curtis N. AZD1152HQPA Accurin™ nanoparticles inhibit growth of diffuse large B-cell lymphomas and small cell lung cancer [abstract]. Proceedings of the 106th Annual Meeting of the American Association for Cancer Research; 2015 Apr 18-22; Philadelphia, PA., 2015. AACR(2015): p. Abstract nr 3102.
- Kerbel RS. Human tumor xenografts as predictive preclinical models for anticancer drug activity in humans: better than commonly perceived-but they can be improved. Cancer Biol Ther. 2003; 2(4 Suppl 1): S134-9.
- Day CP, Merlino G, Van Dyke T. Preclinical Mouse Cancer Models: A Maze of Opportunities and Challenges. Cell. 2015; 163(1): 39-53.
- Song G, Darr DB, Santos CM, et al. Effects of tumor microenvironment heterogeneity on nanoparticle disposition and efficacy in breast cancer tumor models. Clin Cancer Res. 2014; 20(23): 6083-6095.
- Allen TM, Cheng WW, Hare JI, Laginha KM. Pharmacokinetics and pharmacodynamics of lipidic nano-particles in cancer. Anticancer Agents Med. Chem. 2006; 6(6):513-523.
- Cook D, Brown D, Alexander R, et al. Lessons learned from the fate of AstraZeneca’s drug pipeline: a five dimensional framework. Nat Rev Drug Discov. 2014; 13(6): 419-431.
- Rahman M, Akhter S, Ahmad MZ, Ahmad J, Addo RT, Ahmad FJ, Pichon C. Emerging advances in cancer nanotheranostics with graphene nanocomposites: opportunities and challenges. Nanomedicine (Lond). 2015, 10(15), 2405-22.
- Rahman M, Ahmad MZ, Ahmad J, Firdous J, Ahmad FJ, Mushtaq G, Kamal MA, Akhter S. Role of Graphene Nano-Composites in Cancer Therapy: Theranostic Applications, Metabolic Fate and Toxicity Issues.Curr Drug Metab. 2015, 16(5), 397-409.
Issue
Related topics
Formulation, Nano-medicine, Patents, Research & Development (R&D)