High Content Screening for in vitro toxicity testing
Posted: 20 June 2011 |
The application of High Content Screening for in vitro toxicity testing is a relatively new approach in the preclinical research phase of drug development. A battery of tests have been developed for screening on general parameters such as cytotoxicity, while more dedicated assays are available with respect to the identification of genotoxicity, phospholipidosis, steatosis and cholestasis. All these tests are very beneficial within the pharmaceutical industry for the selection of appropriate candidates for drug development as well as for reduction of the attrition rate.
High content screening (HCS) is quickly growing in popularity within the field of in vitro toxicity testing. The maturity of HCS equipment and software has made HCS accessible for many technicians and scientists working in the area of cellular and molecular biology. Although this technique was introduced in the mid 1990’s, the simplification in the use of the software programs, the growth in computer storage capacities as well as the improved qualities of resolution of the digital microscopic cameras has largely increased the accessibility of this equipment. At the start of HCS technology, many scientists were sceptical about this technique as image-based mathematical algorithms had to be written for the analysis.


Figure 1 High content screening analysis of HepG2 cells stained under control (A,C,E,G), or 24 (B,D,F) and 72 (H) h incubations with 10-6 / 10-5 M doxorubicin with different fluorescent probes, i.e.. Hoechst 33342, Fluo-4, TOTO-3 and TMRM
The application of High Content Screening for in vitro toxicity testing is a relatively new approach in the preclinical research phase of drug development. A battery of tests have been developed for screening on general parameters such as cytotoxicity, while more dedicated assays are available with respect to the identification of genotoxicity, phospholipidosis, steatosis and cholestasis. All these tests are very beneficial within the pharmaceutical industry for the selection of appropriate candidates for drug development as well as for reduction of the attrition rate.
High content screening (HCS) is quickly growing in popularity within the field of in vitro toxicity testing. The maturity of HCS equipment and software has made HCS accessible for many technicians and scientists working in the area of cellular and molecular biology. Although this technique was introduced in the mid 1990’s, the simplification in the use of the software programs, the growth in computer storage capacities as well as the improved qualities of resolution of the digital microscopic cameras has largely increased the accessibility of this equipment. At the start of HCS technology, many scientists were sceptical about this technique as image-based mathematical algorithms had to be written for the analysis. However, today many have become enthusiastic about HCS, as HCS can resolve multiple biological issues on the level of individual cells improving the currently used spectrophoto-, fluoro- and luminometric high throughput screening (HTS) assays that only give a mean value of the cell population present per well. The amazing thing with HCS is that you can visualise and score each individual cell for different parameters at multiple wave lengths at a fixed time point. You can even follow biological changes, like uptake, excretion and accumulation, as if you are watching a movie in real time. As all the analytical tools have been pre-programmed or can easily be developed by the suppliers of the equipment, this technology is becoming a standard tool in laboratories for cellular biology.
A variety of HCS instruments are available today1-3. The instruments are used within a broad range of applications, in which the visualisation is based on the allocation of proteins in the cell by tagging the protein of interest with green fluorescence protein or by specific staining with a largely expanding market of specific fluorescent markers or antibodies. In this way, the distribution of e.g. glycoprotein cAMP receptors in the membrane can be monitored and, after activation, their internalisation into the cell can be followed4. Also the relocation of nuclear hormone receptors from the cytosol into the nucleus can be visualised5. Another way is to use HCS in proliferation assays in the area of oncology, in which specific cell growth inhibitors can be identified, such as topoisomerase inhibitors, enzyme inhibitors of the purine, pyrimidine or di- and tetrahydrofolate synthesis or in case of leukemia, breast and prostate tumours by anti-hormones of specific nuclear hormone receptors, like the retinoic acid, oestrogen or androgen receptor.
Also in the field of in vitro toxicology, cellular assays are of particular interest for the early toxicity screening of compounds in the process of drug development within the pharmaceutical industry. While clinical efficacy and ADME were the leading forces for drug failure up to 1990, toxicity and safety issues in preclinical and clinical research became the main causes of attrition in the last two decennia with rates in the order of 30 to 40 per cent6,7. A strategy to overcome these toxicity issues is the implementation of early toxicity screening during the lead optimisation phase with an in vitro screening battery based on HTS and/or HCS8,9. In this review, HCS and in particular the analysis of cytotoxicity, genotoxicity, phospholipidosis, steatosis and cholestasis will be covered. As liver failure is one of the main causes of human toxicity, human and/or rat primary hepatocytes or permanent human liver HepG2 or HepaRG cell lines are commonly used in this respect in combination with permanent Chinese hamster ovary (CHO) or lung (V79) cell lines. Specific examples will be given below. In vitro cytotoxicity testing with HTS has already been performed for a very long time with MEIC, ACuteTox and ToxCast studies10-12. Such tests were also carried out in our laboratory13,14 with a set of 249 compounds using HepG2 cells. Compounds inducing liver necrosis and cell growth inhibition were easily recognised, but many compounds inducing genotoxicity, phospholipidosis, steatosis and cholestasis were missed using these HTS techniques. The sensitivity prediction for in vivo liver toxicity reached a level of 46 per cent (52/113), while selectivity appeared to be very high with 96 per cent (124/130), leading to a predictivity of 73 per cent (182/249). The sensitivity of in vitro cytotoxicity assays can be improved by using HCS, which caused a breakthrough as shown by Peter O’Brien and his colleagues15. They showed that both HepG2 cells and primary hepatocytes can be used for the individual cellular toxicity scoring by microscopy in a 96-well plate. They used relatively simple staining techniques, more or less comparable to HTS. The fluorescent dyes that are commonly used to detect cellular toxicity are: 1) Hoechst 33342 or DAPI, 2) Fluo-4 AM, 3) TMRM or TMRE, 4) TOTO-3 or YOYO-1 and 5) DRAQ5TM for, respectively, the measurement of the DNA content, the intracellular ionised calcium status, the mitochondrial membrane potential, the plasma membrane permeability and the localisation of both the nucleus and cytoplasm. In Figure 1, doxorubicin treatment in HepG2 cells is compared to controls, showing with Hoechst a clear decrease in the cell number, with FLUO-4 AM an accumulation in the cytoplasm and with TOTO-3 in the nucleus, while the mitochondrial membrane potential was completely reduced as shown with TMRM. All these parameters can be analysed simultaneously within the same cell, which is the particular strength of HCS analysis. For 243 drugs with varying degrees of toxicity in vivo, HCS appeared to have a sensitivity of 93 per cent and a specificity of 98 per cent15. These HCS data were supported by Vanparys17 and Abraham et al.18. Vanparys studied 10 compounds, being toxic in the clinic and being non-toxic in vivo in rats, and demonstrated that eight of these compounds could be identified as cytotoxic in HepG2 cells. Abraham et al.18 measured several anti-infective, antilipidemic and antidiabetic drugs, and showed that trovafloxacin, cerivastatin and troglitazone already caused cytotoxic effects in HepG2 cells at dose levels being five to 20-fold lower than those of closely related drugs, being less toxic or even non-toxic in the clinic. The data obtained with HepG2 cells correlated very accurately with the clinical situation, whereas data obtained with primary human and rat hepatocytes only reached a sensitivity of 60 per cent and 70 per cent, respectively19,20. This implies that for in vitro cytotoxicity analysis the HCS cellular observations with HepG2 cells improve the sensitivity of cytotoxicity analysis tremendously towards 93 per cent, whereas with HTS only those compounds influencing cellular proliferation, being approximately 50 per cent of the toxic compounds according to HCS, seem to be classified properly.
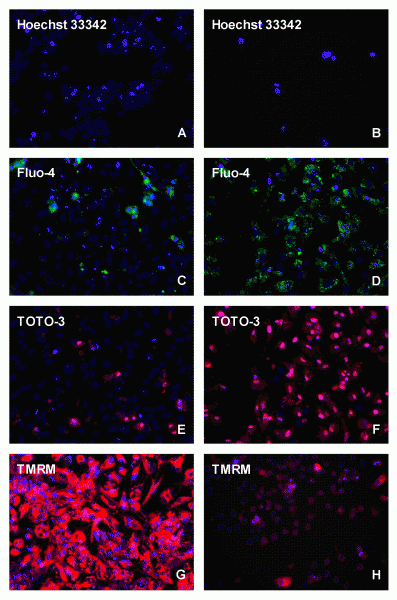
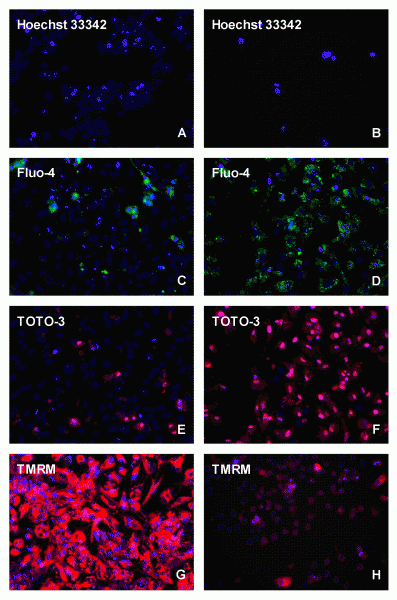
Figure 1 High content screening analysis of HepG2 cells stained under control (A,C,E,G), or 24 (B,D,F) and 72 (H) h incubations with 10-6 / 10-5 M doxorubicin with different fluorescent probes, i.e.. Hoechst 33342, Fluo-4, TOTO-3 and TMRM
For in vitro screening of genotoxicity and carcinogenicity, the FDA and ICH guidelines require, amongst others, an in vitro chromo – somal aberration or in vitro micronucleus assay. In the chromosome aberration test, drug induced irregularities in the chromosomes are determined, whereas in the in vitro micronucleus assay the number of small (micro)-nuclei in close proximity to the nuclear envelopes are counted. When looking at the predictivity of the regulatory approved in vitro genotoxicity assays for in vivo genotoxicity, using a set of 62 ECVAM compounds21,22, a high sensitivity of 100 per cent but a low specificity of 55 per cent is observed (Table 1). The high number of false positive scores in combination with the labour-intensive character of these assays makes them not very suitable as pre-selection tools for early genotoxicity screening. Here HCS screening again can play a pivotal role as with HCS, 50 compounds can easily be screened within one week in the in vitro micronucleus test, whereas with the regular test the maximal throughput reaches only two4. An additional advantage is that with HCS only one to five milligrams of compound is needed23. An automated in vitro micronucleus assay in CHO-K1 has already been described by Diaz et al.24. We compared both CHO-K1 and HepG2 cells with this technique using the 62 ECVAM compounds (Table 1, page 50). The fluorophore Hoechst 33342 was combined with DRAQ5TM for the staining of the nucleus and the cytoplasm and specific algorithms were developed for the segregation of mono – nucleated from binucleated cells and the identification of the micronuclei in those binucleated cells (Figure 2). The specificity scores (Table 1) are much better than with the regular test batteries25. In addition, HTS with HepG2 p53 promoter based luciferase assays score much better in this respect26. The activation of the p53 protein is an early indicator of DNA damage in eukaryotic cells. Moreover, with HCS the activation of p53 can be visualised and quantified with ellipticin27 and doxorubicin (Figure 3). In this case, both HCS and HTS assays are very informative with a highly improved rate of specificity. An additional advantage of HCS is that with this micronucleus test, aneugens can be segregated from clastogens, as large micronuclei only manifest themselves with aneugens, while small micronuclei are seen in case of the clastogens. Moreover, the double strand break biomarker serine 139-phosphorylated histone (γH2AX) assay can be incorporated for the assessment of this specific mechanism of action27,28.
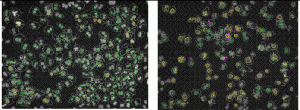
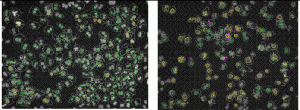
Figure 2 In vitro micronucleus test with CHO-K1 cells under control conditions (A) and in the presence of 10-5 M Taxol (B), showing the identification of many mononucleated (yellow) and binucleated cells (green) as well as many micronuclei ( pink)
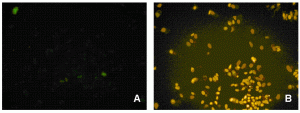
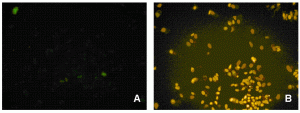
Figure 3 Control (A) and activated (B) status of the p53 protein in CHO-K1 cells
Table 1 Predictive values (%) against in vivo data of the in vitro chromosomal aberration, mouse lymphoma TK, and micronucleus (IVMN) tests23, the HCS – IVMN tests in CHO-K1 and HepG2 cells26, with respect to the ECVAM compound list, including 20 genotoxic and 42 non-genotoxic compounds28 and HepG2 luciferase assays27 (Westerink et al., 2010) and a selection of 56 compounds for phospholipidosis in CHO-K1 and HepG2 cells35.
Assays | Sensitivity | Specificity | Predictivity |
Regular in vitro mammalian genotoxicity tests* ECVAM List |
100.0 |
54.8 |
69.4 |
HCS/HTS genotoxicity tests HCS-IVMN-CHO-K1 HCS-IVMN-HepG2 HTS-Luciferase-HepG2 | 80.0 60.0 85.0 | 88.0 88.0 81.0 | 85.0 79.0 82.0 |
Phospholipidosis tests HCS-PLD-CHO-K1 HCS-PLD-HepG2 | 92.0 88.0 | 87.1 80.6 | 89.3 83.9 |
Phospholipidosis can occur as a hereditary lysosomal storage disorder, like Niemann-Pick disease, but also upon exposure to specific drugs. The excessive accumulation of lipids in the lysosomes occurs most frequently after treatment with cationic amphiphilic drugs with a hydrophobic domain and a hydrophilic side chain. Phospholipidosis is recognised by lamellar bodies in the lysosomes and is often accompanied by the vacuolisation of cells and infiltration of foamy macrophages into tissue. For analysis of phospholipidosis, three fluorophores, Nile Red, LipidTox and NBD-PE, are commonly used29-33. The best signal to noise ratio has been obtained with NBD-PE and an optimal in vitro test can be developed only with flow cytometry or HCS, as for HTS all three fluorophores are too weak for a proper analysis. As shown in Figure 4, upon treatment with the phospholipisosis inducing compound imipramine, NBD-PE accumulates in a concentration dependent way in the cytosol of CHO-K1 cells and more particularly in lysosomes. Analysis with a reference set of 56 compounds revealed very good sensitivity, specificity and predictivity data (Table 1)34. These data are in line with those of flow cytometry31,32 and HCS29,30.
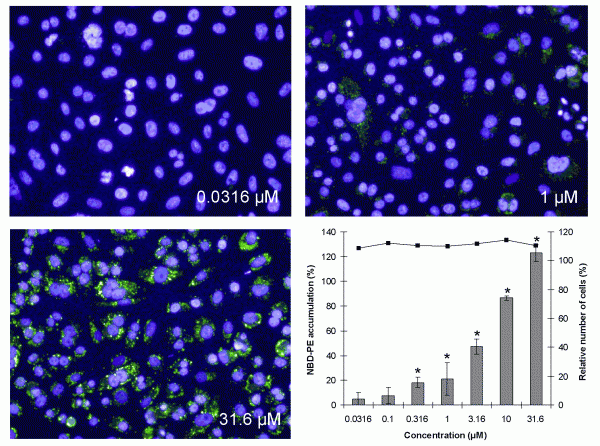
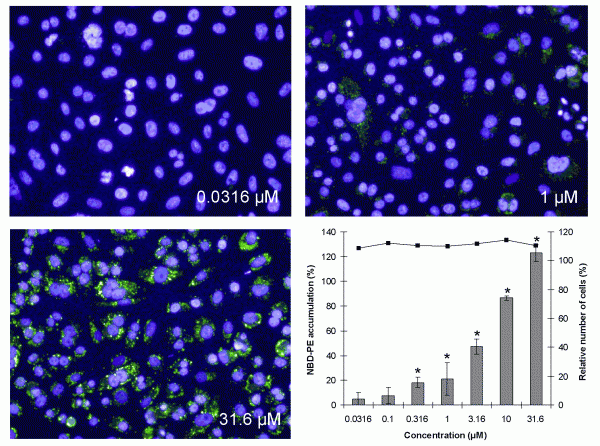
Figure 4 Visual accumulation of NBD-PE in CHO-K1 cells after exposure to 0.0316, 1 and 31.6 μM of imipramine and by calculation of the NBD-PE intensity, showing cell death (solid line) and percentage of fluorescence of NBD-PE compared with the effect of the positive control, 3.16 μM Amiodarone, set at 100 per cent (gray bars) ± SEM. *=significant induction of phospholipidosis (P<0.025)
For the identification of steatosis, Nile Red, Oil red O, and LipidTox were used35,36. To improve the detection of steatosis, fatty acid mixtures can be added to the incubation medium of HepG2 cells, in which a low proportion of palmitic acid (oleate/palmitate (2:1 ratio)) is associated with mild toxic effects, representing a benign chronic steatosis, while a high proportion (oleate/ palmitate (0:3 ratio)) induces an acute and harmful effect by excessive fat accumulation35. As Nile Red and LipidTox are markers that are also used for the analysis of phospholipidosis, a proper discrimination of the mechanism of action with these dyes is hampered. The use of Bodipy 493/503 for steatosis can resolve these problems, as the latter has been shown to be able to discriminate the neutral lipid staining in the cytoplasm from the lysosomal storage effects of the phospholipidotic compounds33,37.
For the assessment of cholestasis it is important to have a hepatocyte culture in which bile canaliculi can develop. Two model systems are described in which primary rat hepatocytes are co-cultured with 3T3 fibroblasts or endothelial cells. In one system, 10 per cent foetal calf serum is added to the culture medium with a reduced oxygen tension ranging from 10 to even three per cent38,39, while in the other system serum was omitted and the oxygen tension was enhanced towards 95 per cent40. Although apparently contra – dictory, both procedures maintain the cytochrome P450 enzyme activities at very high levels and lead to the formation of bile canaliculi. With both procedures, bile canaliculi could be demonstrated by the uptake of 5-(and-6)-carboxy-2′,7′-dichlorofluorescein diacetate (DCF) by the hepatocytes. Within the hepatocytes, DCF is cleaved by esterases, and excreted via ion transporters into the bile canaliculi, which can be visualised by HCS. Cholestatic compounds will prevent this clearance into the bile canaliculi and may therefore lead to a reduced excretion. Another way to examine these ion transporters is to make a sandwich culture41,42 or a monolayer in which the apical uptake versus basal excretion is monitored44. Besides DCF, other dyes, like cholyl-L-lysyl-fluorescein (CLF) and cholylglycylamido- fluorescein (CGamF), are more commonly used to demonstrate disturbances in the bile acid secretion41-44. However, the uptake and excretion of these dyes is relatively fast and further applicability within HTS and HCS has to be established.
Conclusion
A battery of novel in vitro toxicity assays for high content screening has become available for implementation. These novel methods will be of high value in addition to the current regulatory required studies, because they mimic the in vivo biological complexity and predictions in a better way than the currently used HTS, spectrophoto- fluoro- and lumino – metric test systems for cytotoxicity, genotoxicity, phospholipidosis and steatosis. With respect to cholestasis more in depth analysis is needed, but promising tools are presented. Thus if these specific mechanistic tools are included in in vitro toxicity screening procedures, this may have a significant impact on the preclinical development and on the interpretation of the human relevance. In vitro HCS in drug development may have a direct affect on lowering the attrition rate and a reduction in animal use.
Acknowledgements
We would like to thank Masters students Koen Scheerders, Tom Schirris and Jørn Havinga for their assistance with the set-up of the different HCS procedures.
References
- A.H. Gough, P/A. Johnston. Requirements, features, and performance of high content screening platforms. In: High content screening: a powerfull approach to systems cell biology and drug discovery, Eds. D.L. Taylor, J.R. Haskins, K. Giuliano. Humana Press Inc, Totowa, N, USA, Meth. Mol. Biol. Issue 356, Chp 4, pp. 41-61, (2006)
- V. Starkuviene, R. Pepperkok. The potential of highcontent high-throughput microscopy in drug discovery. Br. J. Pharm. Issue 152, pp. 62-71, (2007)
- F. Zanella, J.B. Lorens, W. Link. High content screening: seeing is believing. Tr. Biotech. Issue 28, pp. 237-245, (2010)
- A.F. Hoffman, R.J. Garippa. A pharmaceutical company user’s perspective on the potential of high content screening in drug discovery. In: High content screening: a powerful approach to systems cell biology and drug discovery, Eds. D.L. Taylor, J.R. Haskins, K. Giuliano. Humana Press Inc, Totowa, N, USA, Meth. Mol. Biol. Issue 356, Chp 2, pp. 19-31, (2006)
- S.A. Haney, P. LaPan, J. Pan, J. Zhang. High-content screening moves to the front of the line. DDT Issue 11, pp. 889-894, (2006)
- I. Kola, J. Landis. Can the pharmaceutical industry reduce attrition rates? Nature Reviews Drug Discovery Issue 3, pp. 711-715, (2004)
- F.P. Guengerich, J.S. MacDonald. Applying mechanisms of chemical toxicity to predict drug safety. Chem. Res. Toxicol. Issue 20, pp. 344-369, (2007)
- W.G.E.J. Schoonen, W.M.A. Westerink, G.J. Horbach. High throughput screening for the analysis of in vitro toxicity. Molecular, Clinical and Environmental Toxicology (ed. Andreas Luch). Birkhäuser Publishing, Basel, Switzerland. Issue 1, Chp 14, pp. 401-439, (2009)
- W.G.E.J. Schoonen, W.M.A. Westerink, G.J. Horbach (2010). Application of Medium and High Throughput Screening for in vitro Toxicology in the Pharmaceutical Industry. Alttox Website: http://alttox.org/ttrc/overarching-challenges/wayforward/ schoonen-westerink-horbach/
- C. Clemedson, B. Ekwall. Overview of the MEIC results: I the in vitro – in vitro evaluation. Toxicol. in vitro 13, pp. 657-663, (1999)
- R. Clothier, P. Dierickx, T. Lakhanisky, M. Fabre., M. Betanzos, R. Curren, M. Sjöström, H. Raabe, N. Bourne, V. Hernandez, J. Mainez, M.Owen, S. Watts, R. Anthonissen. A database of IC50 values and principal component analysis of results from six basal cytotoxicity assays, for use in the modelling of the in vivo and in vitro data of the EU ACuteTox project. ATLA Issue 36, pp. 503-519, (2008)
- D.J. Dix, K.A. Houck, M.T. Martin, A.M. Richard, R.W. Setzer, R.J. Kavlock. The ToxCast program for prioritizing toxicity testing of environmental chemicals. Toxicol. Sci. Issue 95, pp. 5-12, (2007)
- W.G.E.J. Schoonen, J.A. de Roos, W.M. Westerink, E. Débiton. Cytotoxic effects of 110 reference compounds on HepG2 cells for compounds on HeLa, ECC-1, and CHO cells. II Mechanistic assays on NAD(P)H, ATP, and DNA contents. Toxicol in vitro Issue 19, pp. 491-503, (2005a)
- W.G.E.J. Schoonen, W.M.A. Westerink, J.A. de Roos, E. Débiton. Cytotoxic effects of 110 reference compounds on HepG2 cells for compounds on HeLa, ECC-1, and CHO cells. I Mechanistic assays on ROS, glutathione depletion and calcein uptake. Toxicol. in vitro Issue 19, pp. 504-516, (2005b)
- P.J. O’Brien, W.Irwin, D.Diaz, E. Howard-Cofield, C.M. Kresja, M.R. Slaughter, B. Gao, N. Kaludercic, A. Angeline, P. Bernardi, P. Brain, C. Cougham. High concordance of drug-induced human hepatotoxicity with in vitro cytotoxicity measured in a novel cellbased model using high content screening. Arch. Toxicol. Issue 80, pp. 580-604, (2006)
- N.T. Lieggi, A. Edvardsson, P.J. O’Brien. Translation of novel anti-cancer cytotoxicity bimarkers with high content analysis from an in vitro predictive model to an in vivo cell model. Toxciol. in vitro Issue 24, pp. 2063-2071, (2010)
- P. Vanparys. New models for toxicity testing. Lecture on 2nd Conference of predictive human toxicity and ADME toxicity studies. 25th-26th January 2007, Brussels, Belgium
- V.C. Abraham, D.L. Towne, J.F. Waring, U. Warrior, D.J. Burns. Application of a high–content multiparameter cytotoxicity assay to prioritize compounds based on toxicity potential in humans. J. Biomolec. Screening Issue 13, pp. 527-537, (2008)
- J.J. Xu. High content screening with primary rat hepatocytes. Lecture Symposium on: High Content Screening for toxicity (Informa). Le Meridian, Vienna, Austria, 28 June 2007
- J.J. Xu, P.V. Henstock, M.C. Dunn, A.R. Smith, J.R. Chabot, D. de Graaf. Cellular imaging predictions of clinical drug-induced liver injury. Tox. Sci. Issue 105, pp. 97- 105, (2008a)
- D.Kirkland, S.Pfuhler, D.Tweats, M.Aardema, R.Corvi, F.Darroudi, A.Elhajouji, H.Glatt, P.Hastwell, M.Hayashi, P.Kasper, S.Kirchner, A.Lynch, D.Marzin, D.Maurici, J.R.Meunier, L.Muller, G.Nohynek, J.Parry, E.Parry, V.Thybaud, R.Tice, J.van Benthem, P.Vanparys, P.White. How to reduce false positive results when undertaking in vitro genotoxicity testing and thus avoid unnecessary follow-up animal tests: Report of an ECVAM Workshop, Mutat. Res. Issue, 628, pp. 31-55, (2007)
- D. Kirkland, P. Kasper, L. Muller, R. Corvi, G. Speit. Recommended lists of genotoxic and non-genotoxic chemicals for assessment of the performance of new or improved genotoxicity tests: a follow-up to an ECVAM workshop. Mutat. Res. Issue 653, pp. 99-108, (2008)
- P. Lang, K. Yerow, A. Nichols, A. Scheer. Cellular imaging in drug discovery. Nature Rev. Issue 5, pp. 343-356, (2006)
- D. Diaz, A. Scott, P. Carmichael, W. Shi, C. Costales. Evaluation of an automatd in vitro micronucleus assay in CHO-K1 cells. Mutat. Res. Issue 630, pp. 1-13, (2007)
- W.M.A. Westerink, A.A. van Staalduinen, J. Polman, G.J. Horbach, W.G.E.J. Schoonen, J.P. Groten. The development and validation of a high content screening in vitro micronucleus assay in CHO-K1 and HepG2 cells. Mutat. Res. (2011)(Accepted)
- W.M.A. Westerink, J.C.R. Stevenson, G.J. Horbach and W.G.E.J. Schoonen. The development of p53, RAD51C, Cystatin A, and Nrf2 luciferase reporter assays in metabolic competent HepG2 cells for the mechanistic genotoxicity and oxidative stress assessment in the early phase of the research. Mutat. Res. Issue 696, pp. 21-40, (2010)
- G.W. Xu, I.M. Mawji, C.L. Macrae, C.A. Koch, A. Datti, J.F. Wrana, J.W. Dennis, A.D Schimmer. A high-content chemical screen identifies ellipticine as a modulator of p53 nuclear localization. Apoptosis Issue 13, pp. 413- 422, (2008b)
- P.R. Barber, R.J. Locke, G.P. Pierce, K. Rothkamm, B. Vojnovic. Gamm-H2AX foci counting image processing and control software for high contentscreening. In: Imaging , manipulation, and analysis of biomolecules, cells and tissues V. (Eds. D.L. Farkas, R.C. Leif, D.V. Nicolau, Proc. of SPIE, Issue 6441, pp. 1M1-10, (2007)
- P. Nioi, B.K. Perry, E.-J. Wang, Y.-Z. Gu, R.D. Snyder. in vitro detection of drug-induced phospholipidosis using gen expression and fluorescent phospholipid-based methodologies. Tox. Sci. Issue 99, pp. 162-173, (2007)
- J.K. Morelli, M. Buehrle, F. Pognan, L.R. Barone, W. Fieles, P.J. Ciacco. Validation of an in vitro screen for phospholipidosis using a high-content biology platform. Cell Biol. Toxicol. Issue 22, pp. 15-27, (2006)
- N. Mesens, M. Steemans, E. Hansen, A. Peters, G. Verheyen, P. Vanparys. A 96-well flow cytometric screening assay for detecting in vitro phospholipidosis-induction in the drug discovery phase. Toxicol. in vitro Issue 23, pp. 217-226, (2009)
- N. Mesens, M. Steemans, E. Hansen, G. Verheyen, F. van Goethem, J. van Gompel. Screening for phospholipidosis induced by central nervous drugs: comparing the predicitivity of an in vitro assay to high throughput in silico assays. Toxicol. in vitro Issue 24, pp. 1417-1425 (2010)
- M. Grandl, G. Schmitz. Fluorescent high content imaging allows the discrimination and quantitation of E-LDL-induced lipid droplets and Ox-LDL-generated phospholipidosis in human macrophages. Cytometry Issue 77A, pp. 231-242, (2010)
- F. van de Water, J. Havinga, W.T. Ravesloot, G.J. Horbach, W.G.E.J. Schoonen. High content screening analysis of phospholipidosis: validation of a 96-well assay with CHO-K1 and HepG2 cells for the prediction of in vivo based phospholipidosis. Toxicol. in vitro, (Submitted)
- M.J. Gómez-Lechón, M.T. Donato, A. Martínez-Romero, N. Jiménez, J.V. Castell, J.-E. O’Connor. A human hepatocellular in vitro model to investigate steatosis. Chemico-Biological Interactions Issue 165, pp. 106-116, (2007)
- J.G. Granneman, H.-P. H. Moore, E.P. Mottillo, Z. Zhu. Functional interactions between Mldp (LSDP5) and Abhd5 in the control of intracellular lipid accumulation. JBC Issue 284, pp. 3049-3057, (2009)
- M.T. Donato, A. Martínez-Romero, N. Jiménez, A. Negro, G. Herrera, J.V. Castell, J.-E. O’Connor, M.J. Gómez-Lechón. Cytometric analysis for drug-induced steatosis in HepG2 cells. Chemico-Biological Interactions Issue 181, pp. 417-423, (2009)
- J.W. Allen, S.R. Khetani, S,N, Bhatia. in vitro zonation and toxicity in a hepatocytes bioreactor. Tox. Sci. Issue 84, pp. 110-119, (2005)
- S.R. Khetani, S,N, Bhatia. Microscale culture of human liver cells for drug development Nature Biotechnol. Issue 26, pp. 120-126, (2008)
- S. Kidambi, R.S. Yarmush, E. Novik, P. Chao, M.L. Yarmush, Y. Nahmias. Oxygen-mediated enhancement of primary hepatocytes metabolism, functional polarization, gene expression, and drug clearance. PNAS Issue 106, pp. 15714-15719, (2009)
- Z.-W. Ye, P. Augustijns, P. Annaert. Cellular accumulation of cholyl-glycylamido-fluorescein in sandwich-cultured rat hepatocytes: Kinetic characterization, transport mechanisms and effect of human immunodeficiency virus protease inhibitors. DMD Issue 36, pp. 1315-1321, (2008)
- Z.-W. Ye, J. van Pelt, S. Camus, J. Snoeys, P. Augustijns, P. Annaert. Species-specifc interaction of HIV protease inhibitors with accumulation of cholyl-glycylamidofluorescein (CGamF) in sandwich-cultured hepatocytes. J. Pharm. Sci. Issue 99, 2886-2898, (2010)
- S. Mita, H. Suzuki, H. Akita, H. Hayashi, R. Onuki, A.F. Hofmann, Y. Sugiyama. Inhibition of bile acid transport across Na+/taurocholate cotransporting polypeptide (SLC10A1) and bile salt export pump (ABCB 11)- coexpressing LLC-PK1 cells by cholestasis-inducing drugs. DMD Issue 34, pp. 1575-1581, (2006)
- D.R. de Waart, S. Häuser, M.L.H. Vlaming, C. Kunne, E. Hänggi, H.-J. Gruss, R.P.J. Oude Elferink, B. Stieger. Hepatic transport mechanisms of cholyl-L-lysylfluorescein. JPET Issue 334, pp. 78-86, (2010)