Continuous manufacturing: regulatory and quality assurance challenges
Posted: 22 August 2017 | Dave Elder, Ronak Savla, Stephen Tindal | No comments yet
During 2002, the United States Food and Drug Administration (FDA) requested rationalisation and modernisation of the manufacturing base for pharmaceutical production. This was in the hope that modernising the supply chain would enhance the robustness of manufacturing processes, thereby reducing product failures and, importantly, significantly enhancing product quality.1 The FDA’s recommendations were to encourage the early adoption of technological advances by the industry, and to facilitate industry application of modern quality management techniques to all aspects of production and quality assurance.
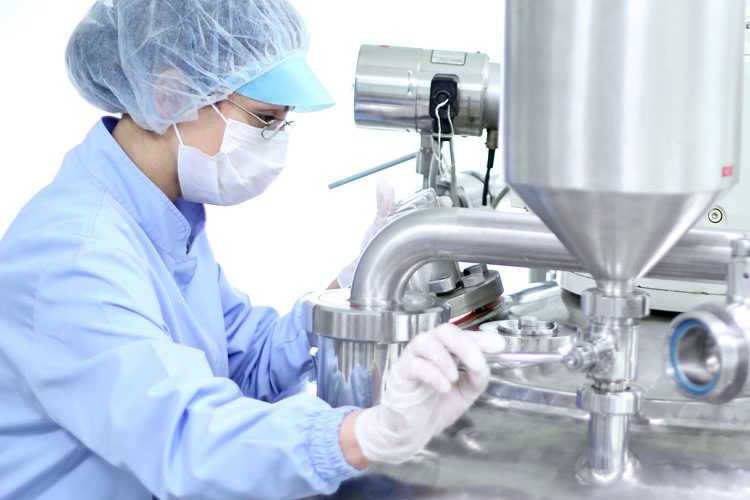
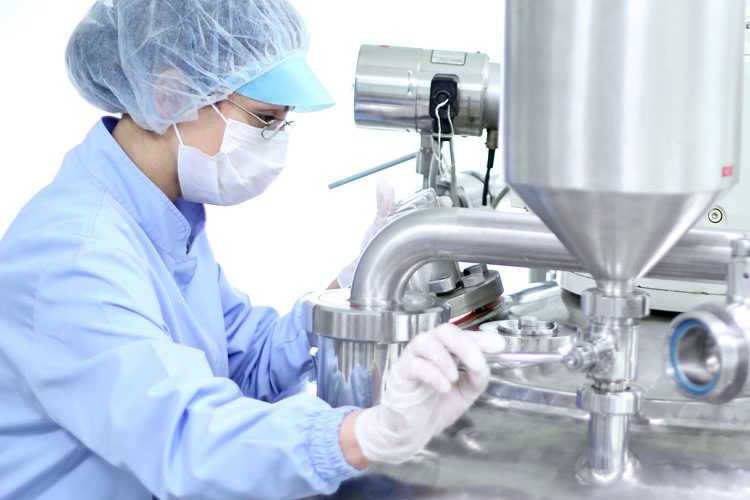
In addition, the FDA recognised that adoption of innovative manufacturing approaches could present significant challenges, not least in the adoption of appropriate pharmaceutical quality systems (PQS). There were concerns within the pharmaceutical industry that implementation of these emerging technologies could penalise ‘early adopters’ and result in delays to the proposed submission. As a consequence, the FDA initiated the Emerging Technology Team (ETT) to embed awareness into the agency2 and has subsequently held a significant number of ETT-industry meetings since its inception in 2014. ETT-industry meetings routinely discuss API and drug product applications, as well as the control strategies that are being considered.3
The European Medicines Agency (EMA) was also a strong advocate of the need for industry to change and modernise and it updated its current regulatory framework to support the introduction of continuous manufacturing.4 However, the agency recognised the challenges and noted that continuous manufacturing dossiers would likely be more complex, thus recommending early dialogue between it and manufacturers, especially for legacy products where the intention is to transfer from existing batch manufacture to the preferred continuous manufacturing. The EMA promised to support industry via scientific advice, process analytical technology (PAT) teams, subject matter expert (SME) advice, and the implementation of an Innovation Task Force (ITF).5
Thus, both agencies advocated that, in parallel with adoption of these new innovative technologies, there was a need for a different and improved approach to quality management within the industry. This in turn was one of the catalysts for the development and implementation of ICH Q10: Pharmaceutical Quality System.6 ICH Q10 defines a comprehensive model for an effective PQS based on existing internationally accepted quality standards, ie, those of the International Standards Organization (ISO).7
ICH Q10 includes the applicable current Good Manufacturing Practice (cGMP) regulations and is designed to complement ICH Q8: Pharmaceutical Development8 and ICH Q9: Quality Risk Management9 guidelines. Importantly, ICH Q10 seeks to identify a PQS model that can be implemented throughout the different stages of a product’s lifecycle, in addition to the manufacture of commercial products. Therefore, the majority of ICH Q10 is applicable to existing manufacturing sites, which are controlled by regional cGMP requirements. ICH Q10 is therefore intended to support an effective PQS that should enhance both the quality and availability of medicinal products on a global basis. Finally, implementation of ICH Q10 across a product’s lifecycle and during production is intended to facilitate continuing innovation and continual improvement and to strengthen the connectivity between ongoing pharmaceutical development and the requisite manufacturing activities.6
Continuous processing
Continuous manufacturing was one of the primary new technologies that regulatory agencies hoped industry would introduce. This approach offers opportunities for significant reduced costs while improving robustness and quality, and thereby reducing product failures. In continuous manufacturing, the input materials are constantly introduced with product being discharged from the system, throughout the duration of the process. Thus, the output from each individual processing step travels continuously forward to the following sequential processing step without a holding time. The overall process speed is defined by the rate of the slowest individual processing step. Labelling of each material at the end of the process necessarily involves some aspect of timestamping (instead of traditional batch numbering) in order to maintain traceability.
Batch and batch release are both important parameters in any continuously operated process. A batch can be defined as having ‘uniform character and quality within specified limits’.10 The size of the batch can also be defined based on quality, quantity or time-based criteria. Indeed, if an appropriate state of control can be shown, then the batch definition could include large quantities of material, even if different processing conditions or different lots of input materials have been used.11 Conversely, there may be periods where the process is ‘out of control’ and the control system needs to have the flexibility and responsiveness to allow this material to be designated as sub-optimal and diverted to waste.
Additionally, the process needs to be responsive to any product quality issues associated with any of the input raw materials, customer complaints, product recalls, etc.12 This requires an in-depth understanding of material flow within the process, thereby ensuring that affected material can be tracked using appropriate in-line or at-line monitoring, ie, process analytical testing (PAT), and diverted if required. Therefore, adequate traceability of all input materials is imperative, allowing a complete reconstruction of the batch history if required, ie, total material traceability.
The key vulnerabilities of any continuous process are obviously the start-up and shut-down points, when the state of control may either not have been established or where there is no longer a state of control. Thus, the start point of the batch will not be the same as the start-up point of the process, but that point where the process is under control as defined by pre-defined criteria such as critical material attributes (CMAs), critical process attributes (CPPs) and/or critical quality attributes (CQAs) all being within pre-defined limits. The same approach can be taken to shut-down, and the end of the process needs to be appropriately defined. These key parameters will normally be evaluated during development and confirmed during process verification.
Quality/cGMP considerations
Typically, a change from a batch to a continuous processing operation will necessitate changes to the facility (or manufacturing area), changes to equipment, process parameters and the control strategy.12 Therefore, the site needs to assess its overall PQS and supporting processes to ascertain what changes are required and whether these changes have any impact on existing batch-based processes. The risk assessment needs to take an overarching view of the proposed changes, ie, batch versus continuous manufacturing.9,11 Factors such as the proposed manufacturing process, eg, wet or dry granulation versus direct compression, drug loading (high versus low), drug potency (high versus low), etc, can significantly impact drug product quality. For example, the risk of an immediate-release product with high drug loading would be reduced if there was extensive experience and knowledge of the API physicochemical properties and underlying process capability.
However, if there were plans to incorporate dual-sourcing of the API, or to use a different API supplier, then the risks could be greater. It is important to engage in dialogue with the appropriate regulatory agencies before implementing any changes.12 The sponsor can delineate the reason for the proposed change, eg, increased supply chain security, increased efficiency or decreased costs, etc. The impact on product CMAs, CPPs, CQAs and finally the impact on the bioavailability of the two products and whether a biowaiver or a bioequivalence study is required, should also be considered.1
Technology transfers should be less challenging than for comparative batch-based processes, even with the increased challenges of greater complexity and up-skilling of production personnel. This is because the process can often be run at the same or similar scale as in R&D, the processes just being run for longer periods. If scale-up is required, it is easier to accomplish using continuous processing than classical batch processing.13,14 It is imperative that adequate expertise of the new technology is embedded within the manufacturing and quality organisations.
There are concerns regarding the availability of experienced staff with sufficient expertise to run continuous processes in production settings.15 This is primarily because new skill sets are required for continuous manufacturing, ie, statisticians, chemometricians and engineers.16 It is also important to ensure suitable up-skilling of existing staff to ensure they can cope with the demands of the new technologies. Specific responsibilities within both quality and manufacturing functions should be defined, and this can have ramifications on the quality culture within the site. For example, it should be ascertained who is responsible for stopping (or re-starting) continuous operations, how non-conforming materials are documented, and whether existing CAPA (corrective action and preventive action) processes need to be modified to address the introduction of the new technology.11 Quality personnel will also require full training and an understanding of statistical process control systems, as these approaches play a more significant role in continuous processing than in existing batch processing (see ‘Real-time release testing’, below).
Focus on key input materials
From a quality perspective, there needs to be greater focus and subsequent control over key input materials.12 Continuous manufacturing may require extra ‘functionality’ tests for the API release specification. In addition, if continuous manufacturing is being considered as part of life-cycle management to reduce costs and/or increase capacity, then the API supply chain may already have been diversified. In these scenarios the continuous process needs to be robust enough to cope with likely differences in the physical properties of the input API. Fonteyne et al17 showed that the material properties of theophylline from seven different suppliers varied and impacted on processability/CQAs of the resultant granules and tablet, when using a continuous manufacturing process.
Similarly, for batch-based processes excipients are tested for compliance with the existing pharmacopoeial monograph and there is typically little need for additional functionality tests to ensure the material is fit for purpose. In contrast, for continuous manufacturing, material variability (batch-to-batch, supplier-to-supplier) is critically important, and additional tests may be required. Even well established, pharmacopoeial grade excipients, eg, microcrystalline cellulose (MCC) can exhibit differences in their CMAs.
A systematic, blinded study of six batches of MCC from the same supplier was performed by Fonteyne et al.18 They showed differences in particle size distribution and crystallinity, which adversely affected granule size distribution in a continuous wet granulation process. Differences in CMAs can affect material throughput in any continuous manufacturing process. Additional excipient functionality tests must therefore be carried out using spectroscopy based techniques, eg, Raman or near infrared, which can provide fast data turnaround to allow disposition decisions to be made rapidly.19-21
Cleaning and cleaning validation are important considerations for any continuous processing operation.22 If the process uses dedicated equipment then carry-over and potential contamination of any subsequent product will not be an issue. However, it is best practice to perform a risk assessment to fully understand the potential problems.9 Potential issues include microbiological quality and the potential for carry-over of degradation products or cleaning agents from one ‘batch’ to the next. In addition, cleaning frequency may need to be more accurately defined than for standard batch processes. For instance, the cleaning interval could be defined based on:
- Incidence of any process deviations or CAPAs
- Total quantity of product (or material processed)
- Elapsed time
Some of the cleaning approaches that can be employed include:
- In-process cleaning of critical PAT-based equipment, eg, sensors
- Better design of sensors to prevent fouling
- Single-use sensors in biopharmaceutical applications
- Stopping production, or diverting to waste output material if critical equipment, sensors or analysers require cleaning
- Employing a second process train while the first is taken off-line and cleaned.11,22
Risk assessment, modelling and testing are required to assess the different vulnerabilities of the continuous manufacturing apparatus
Similar considerations apply to planned maintenance or break-down of critical equipment, sensors or analysers. Risk assessment, modelling and testing are required to assess the different vulnerabilities of the continuous manufacturing apparatus.9 These assessments could conclude that planned redundancy, duplication, or the installation of parallel arrays are required. Procedures should be in place in case of breakdown of PAT-based sensors that will allow another approach, ie, alternative continuous monitoring using different sensors or the use of end-product testing.11
Regulatory overview
There are certain differences in the regulatory expectations for continuous manufacturing, which are centred on the risk assessment process.9 These include:
- Risks and control strategies are likely to be different from a standard batch process
- Model-based control systems are frequently employed, involving multivariate monitoring, the analysis of very large data sets, elements of automation and PAT that can potentially result in real-time release testing (RTRT)
From a regulatory perspective, some of the key challenges of continuous manufacturing are:
- The need for enhanced process understanding.23 The availability and implementation of mechanistic models that are applicable to all of the processing steps, together with the application of multivariate assessment of product quality.
- Adequate and representative allocation of PAT sensor probes to ensure adequate sampling frequency.14
- Linking the various on-line PAT monitoring systems to the control system to facilitate this feedback/feed-forward control strategy still presents a significant challenge.14
- Global regulatory divergence on continuous manufacturing was recently cited by Pfizer as one of the main impediments to successfully applying continuous technology.24
Control strategy
The capacity to identify, separate and reject non-conforming materials in real-time (or near real-time) is one of the critical aspects of any continuous manufacturing control strategy.10 The assessment and subsequent understanding of the reasons for these deviations, the amount of the total batch impacted, and the rationalisation for any remediation, are critically important. Therefore, process control systems require the following attributes:
- Rapid and controlled start-up and shutdown procedures.
- Continuous compliance of all CMAs/CQAs/CPPs by rapidly achieving a steady state condition.
- A total assurance of quality, irrespective of any system perturbations.25 This in turn necessitates various feedback, feed-forward control measures, etc, and the ability to trace OOT (out of trend) and OOS (out of specification) material or process inputs to specified finished material.
Appropriate placement of PAT sensors is often a key element of the control strategy.25 PAT monitoring of indirect product performance measures may also be required, eg, indirect measure of changes in granulation end-point using mixer impellor power consumption or torque.26 Advances in automated process control systems that model predictive control approaches are still required.12
Real-time release testing (RTRT)
RTRT is a fundamental part of any control system for the continuous process. Process parameters can then be automatically modified to ensure conformance with the established acceptance criteria.10 RTRT can be applied during any stage of an API or drug product.27 Sometimes, RTRT can be based on an indirect measure of product performance. Singh et al28 recently reported on real-time monitoring of the bulk density of a powder blend in a continuous direct compaction tabletting process. This was coupled to feed-forward and feedback controls. It is obviously desirable to have adequate on-line/at-line PAT measurements such that off-line analysis is not required. However, the reality is that the sensitivity and selectivity of spectroscopically based PAT tools are not currently aligned with existing ICH Q3A29/Q3B30 impurity expectations.14
The increased frequency of data collection should result in a higher quality product,31 but it also necessitates the application of statistical methodology for these larger sample sizes, which are required to enhance confidence that the batch conforms to the required quality.32 However, the use of ‘big data’ also presents data integrity challenges. In addition, real-time release also necessitates real-time storage of all relevant processing data.13 Furthermore, the likelihood of observing OOS results is heightened from a statistical perspective when large volumes of data are generated. Additionally, sampling frequency needs to be aligned with on-line PAT capability.25
Conclusion
Modernising the manufacturing supply chain leads to obvious benefits for the industry. However, the regulatory and quality challenges are often downplayed. Lack of global regulatory harmonisation and understanding often means that manufacturing sites must run old and new processes side by side. The complete implementation of any novel technology is driven by the speed of the slowest global regulatory approval, not the fastest.33
Continuous manufacturing often requires manufacturing sites to re-appraise their QMS and employ new personnel with aligned skillsets, while upskilling existing staff. In addition to greater understanding of material attributes there needs to be enhanced functionality testing of API and excipients. In parallel, there is significantly enhanced continuous testing of the process via in-line/at-line PAT sensors/probes. Given the challenges of data integrity for existing batch processes, ‘big data’ presents huge challenges within the production environment. Lastly, robust control strategies require near real-time decision making, which can present cultural challenges.
About the authors
References
- FDA, 2002. Pharmaceutical cGMPS for the 21st Century – A Risk-Based Approach: Second Progress Report and Implementation Plan.
- FDA, 2015. Advancement of emerging technology applications to modernize the pharmaceutical manufacturing base. Guidance for Industry. Centre for drug evaluation and research (CDER), Pharmaceutical quality/CMC.
- Lee SL, O’Connor TF, Yang X, Cruz CN, Chatterjee S, Madurawe RD, Moore CMV, Yu LX, Woodcock J. Modernizing Pharmaceutical Manufacturing: from Batch to Continuous Production. J Pharm Innov. 2015;10(3):191-199.
- Hernan D. Continuous manufacturing: challenges and opportunities: EMA perspective. 3rd FDA/PQRI Conference on Advancing Product Quality. March 22-24, 2017.
- EMA, 2014. Mandate of the EMA Innovation Task Force (ITF). EMA/484400/2014, Human Medicines Research and Development Support Division.
- ICH Q10, 2008. Pharmaceutical quality system. Current step 4 version, dated 04 June 2008.
- International Organization for Standardization (ISO), 2017. https://www.iso.org/standards.html. Accessed on 14 August 2017.
- ICH Q8(R2). 2009. Pharmaceutical development. Current step 4 version, August 2009.
- ICH Q9. 2005. Quality Risk Management. Current step 4 version, 09 November 2005.
- Lee SL. Current FDA perspective for continuous manufacturing. MIT-CMAC 2nd International Symposium on Continuous Manufacturing of Pharmaceuticals. September 26-27, 2016.
- Allison G, Cain YT, Cooney C, Garcia T, Bizjak TG, Holte O, Jagota N, Komas B, Korakianiti E, Kourti D, Madurawe R, Morefield E, Montgomery F, Nasr M, Randolph W, Robert J-L, Rudd D, Zezza D. Regulatory and quality considerations for continuous manufacturing May 20-21, 2014 Continuous manufacturing symposium, J. Pharm. Sci. 2015;104:803-812.
- Lee SL, O’Connor TF, Yang X, Cruz CN, Chatterjee S, Madurawe RD, Moore CMV, Yu LX, Woodcock J. 2015. Modernizing pharmaceutical manufacturing: From batch to continuous production. J Pharm Innov. 2015;10(3):191-199.
- Page T, Dubina H, Fillipi G, Guidat R, Patnaik S, Poechlauer P, Shering P, Guin M, Jonston C. Equipment and analytical companies meeting continuous challenges May 20-21, 2014 Continuous manufacturing symposium, J Pharm Sci. 2015;104: 821-831.
- Baxendale IR, Braatz RD, Hodnett BK, Jensen KF, Johnson MD, Sharratt P, Sherlock J-P. Florence AJ. Achieving continuous manufacturing: Technologies and approaches for synthesis, workup and isolation of drug substance May 20-21, 2014 Continuous manufacturing symposium. J Pharm Sci, 2015;104:781-791.
- Poechlauer P, Manley J, Broxterman R, Gregertsen B, Ridemark M. Continuous processing in the manufacture of active pharmaceutical ingredients and finished dosage forms: An industry perspective. Org Proc Res Dev. 2012;16:1586-1590.
- Nepveux K, Sherlock J-P, Futran M, Thien M, Krumme M. How development and manufacturing will need to be structured – Heads of development/manufacturing. May 20-21, 2014. Continuous manufacturing symposium. J Pharm Sci. 2015;104:850-864.
- Fonteyne M, Wickström H, Peeters E, Vercruysse J, Ehlers H, Peters B-H, Remon JP, Vervaet C, Ketolainen J, Sandler N, Rantanen J, Naelapää K, De Beer T. Influence of raw material properties upon critical quality attributes of continuously produced granules and tablets. Eur J Pharm Biopharm. 2014;87:252-263.
- Fonteyne M, Correia A, De Plecker S, Vercruysse J, Ilic I, Zhou Q, Vervaet C, Remon JP, Onofre F, Bulone V, De Beer T. Impact of microcrystalline cellulose material attributes: A case study on continuous twin screw granulation. Int J Pharm. 2015;478:705-717.
- Paudel A, Raijada D, Rantanen J. Raman spectroscopy in pharmaceutical product design. Adv Drug Deliv Rev. 2015;89:3-20.
- Esmonde-White KA, Cuellar M, Uerpmann C, Lenain B, Lewis IR. 2017. Raman spectroscopy as a process analytical technology for pharmaceutical manufacturing and bioprocessing. Anal Bioanal Chem. 2017;409(3):697-649.
- Tomută I, Iovanov R, Bodoki E, Leucuta SE. 2010. Quantification of Meloxicam and excipients on intact tablets by near infrared spectrometry and chemometry. Farmacia. 58(5):559-571.
- Elder DP (moderator). In-depth focus: PAT roundtable. Eur Pharm Rev. 2015; 20(6), 57-59.
- Chatterjee S. FDA perspective on continuous manufacturing. IFPAC annual meeting, Baltimore, January 2012. www.fda.gov/downloads/aboutfda/centersoffices/officeofmedicalproductsandtobacco/cder/ucm341197.pdf. Accessed on 14 August 2017.
- Stanton D. 2017. Pfizer: Global regulatory divergence restricted continuous manufacturing ambition. www.in-pharmatechnologist.com/Processing/Pfizer-on-continuous-manufacturing-and-regulatory-divergence. Accessed on 14 August 2017.
- Rantanen J, Khinast J. The future of pharmaceutical manufacturing sciences. J Pharm Sci. 2015;104:3612-3638.
- Narang AS, Sheverev VA, Stepaniuk V, Badawy S, Stevens T, Macias K, Wolf A, Pandey P, Bindra B, Varia S. 2015. Real-time assessment of granule densification in high shear wet granulation and application to scale-up of a placebo and brivanib alaninate formulation. J Pharm Sci. 2015;104:1019-1034.
- Singh R, Román-Ospino AD, Romañach RJ, Ierapetrtou M, Ramachandran R. Real time monitoring of powder bulk density for coupled feed-forward/feedback control of a continuous direct compaction tablet manufacturing process. Int J Pharm. 2015;495:612-625.
- Elder DP. 2017. An overview of process analytical control. Eur Pharm Rev. 2017;20(6).44-47.
- ICH Q3A(R2). 2006. Impurities in new drug substances. Current step 4 version, 25 October 2006.
- ICHQ3B(R2). 2006. Impurities in new drug products. Current step 4 version, 02 June 2006.
- Byrn S, Futran M, Thomas H, Jayjock E, Maron N, Meyer RF, Myerson AS, Thien MP, Trout BP. 2015. Achieving continuous manufacturing for final dosage formation: Challenges and how to meet them May 20-21, 2014 Continuous manufacturing symposium. J Pharm Sci. 2014;104:792-802.
- Schilling EG, Neubauer DV. 2009. Acceptance sampling in quality control. Boca Raton, 2nd Edition, CRC Press.
- Shanley AQ. Breaking through the red tape to modernize manufacturing. Pharm Tech. Eur. 2017;29(8):10-14.
Issue
Related topics
Drug Manufacturing, Manufacturing, QA/QC, Regulation & Legislation