Stem cell nano drug delivery applications to treat diabetes
Posted: 4 May 2016 | Anjali Hirani (University of South Florida Health), Vishal Shahidadpury (University of South Florida Health), Yashwant Pathak (University of South Florida Health) | No comments yet
Stem cell therapy is currently utilised in medicine for its application in tissue repair and regenerative medicine. Unique characteristics of stem cells, such as self-renewal and differentiation, allow its function to be applicable for therapeutic purposes. Nanotechnology and cell microencapsulation have been researched and used as a means to protect cells from innate immune responses while permitting targeted drug delivery, as well as for sustained release of therapeutic agents to specific organs or cells. This review will provide an overview of stem cells, the purpose of nanotechnology and various surface modifications of nanoparticles for enhanced drug delivery, as well as describe how stem cell therapy is used for type 1 and type 2 diabetes mellitus. Additionally, a specific application of nanoparticle encapsulation and human embryonic stem cells in achieving glycaemic control, as seen in type 1 diabetes-induced immune-competent mice, will be discussed…
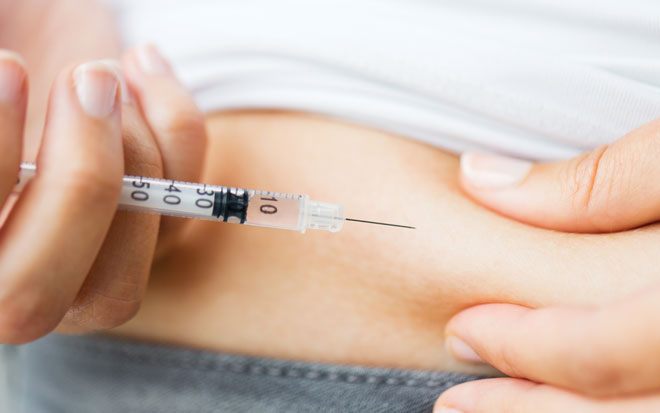
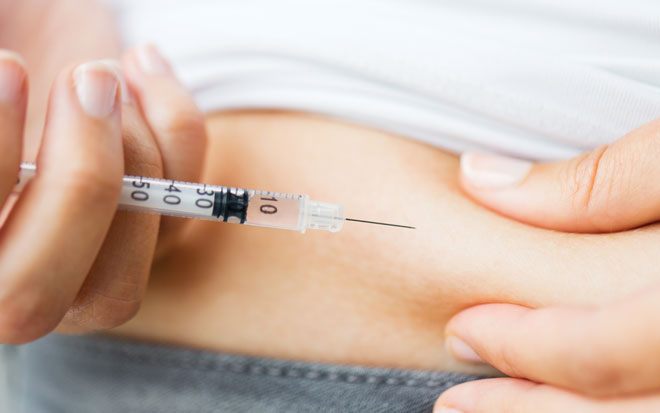
Introduction
Stem cells are found in nearly all tissues throughout the body and are characterised by the notable attributes of self-renewal and differentiation capacity1. Self-renewal is defined as the cell’s ability to undergo cycles of mitotic division while keeping the parent cell’s same undifferentiated state2. Stem cells can be derived from embryonic and adult tissues and subsequently categorised into pluripotent and multi-potent cells, respectively. A stem cell’s differentiation potential is closely dependent on its origin3. A pluripotent stem cell is defined by its capacity for indefinite self-renewal1 . Included under the pluripotent cell category are embryonic stem cells (ESCs) and induced pluripotent stem cells (iPSCs).
ESCs are derived from the inner cell mass of the pre-implantation blastocyst. They are able to give rise to all cell lineages of the three embryonic germ layers: the endoderm, ectoderm and mesoderm, and are thus considered pluripotent cell types3. iPSCs are obtained by reprogramming mature somatic cells to a pluripotent state via small sets of determined genetic factors, and therefore provide major implications in human genetics and regenerative medicine4.
Multipotent stem cells differ from pluripotent stem cells in that they are more restricted in their capacity for differentiation. Common examples of multipotent stem cells include mesenchymal stem cells (MSCs) and haematopoietic stem cells (HSCs). MSCs are obtained from multiple sources including adipose tissue, bone marrow, umbilical cord tissue and amniotic fluid. They can be isolated from the perivascular compartment of most organs including the liver and are able to differentiate to the mesodermal lineage including bone, cartilage and fat4.
Adult MSCs have been utilised for regenerative purposes in vivo since 1950. Since this time they have been used to restore tissue functionality in various malignancies including Parkinson’s disease, Alzheimer’s disease, cardiovascular diseases and other degenerative disorders affecting muscles, lung, liver, tendons and other organs3. Haematopoietic stem cells are obtained from bone marrow and umbilical cord blood. They too have been widely utilised in stem cell therapy. HSC transplantation has been regarded as the most successful application of stem cells in medicine; it can be used to treat genetic blood disorders such as thalassemia and immune deficiency, as well as ailments including leukaemia and lymphoma5.
In medicine both MSCs and HSCs are being used for patients with diabetes or liver diseases, including acute liver failure, fulminant hepatitis and inborn errors of metabolism, hepatitis viruses, liver toxins, alcohol consumption, autoimmunity and metabolic disorders such as non-alcoholic steato-hepatitis. Stem cell therapy offers a promising future of potential therapies and regenerative medicine strategies for patients4.
Recent trends in nanotechnology
Nanoparticles in the range of 100nm in size are solid, spherical structures synthesised from natural or synthetic polymers. A diverse quantity of drugs can be delivered via nanoparticles, including hydrophilic and hydrophobic drugs, vaccines and biological macromolecules. These tiny particles allow the targeted administration of therapeutics, or controlled drug delivery, to specific organs and cells6. They are useful for delivering concentrated doses to target locations with extended circulation times and increased uptake7. Poly-lactic-co-glycolic acid (PLGA) is a biodegradable polymer used in nanoparticle preparation. Its metabolites, lactic acid and glycolic acid are removed easily from the body via the Krebs cycle. PLGA nanoparticles can be taken up by cells through pinocytosis and also through endocytosis.
Hydrophobic particles are identified as foreign to the body and are thus eliminated from the blood stream via the reticulo-endothelial system. Nanoparticles can be coated with molecules that conceal their hydrophobic nature by providing a hydrophilic layer on the surface. Most commonly, PLGA nanoparticles are able to achieve hydrophilicity by the addition of the non-ionic polymer, polyethylene glycol (PEG)6. It has been seen that PEGylation demonstrates a significantly decreased uptake by macrophage phagocytic cells, thus preserving the bioactivity of the encapsulated therapeutic agent8.
Chitosan and Poloxamer have also been previously studied for surface modification. These polymers obstruct electrostatic and hydrophobic interactions, which support the binding of opsonins to particle surfaces. Surface charges also remain a contributing factor to the successful internalisation of nanoparticles in cells. Previous studies show that a positively-charged nanoparticle will allow a higher extent of internalisation due to the interaction of the positively-charged nanoparticle and the negatively-charged cell membrane. PLGA nanoparticles have negative charges, which can be altered to neutral or positive charges through surface modification via PEGylation or chitosan coating6.
Overall, nanotechnology is beneficial in the application of targeting specific organs and cells, its receptivity to external stimuli, imaging capabilities and drug delivery7.
Application of nanotechnology
Diabetes mellitus is the most common endocrine disease in the world and is an important factor in mortality and morbidity. The number of people with diabetes has increased in recent years. Type 1 diabetes mellitus (T1DM) is an autoimmune disease characterised by an absolute deficiency of the secretion of insulin due to inflammation, necrosis or apoptosis of pancreatic islet β cells9 and accounts for less than 10% of patients with diabetes. T1DM develops in childhood and may lead to long-term complications including retinopathy, neuropathy, nephropathy, as well as macrovascular diseases, which include cerebral, coronary or peripheral vascular systems10. Studies have shown that the autoimmune destruction of β cells in T1DM diminishes a person’s ability to regulate blood glucose, eventually resulting in poor blood circulation, heart disease, stroke, infection, kidney failure and premature death11.
Most (90%) pf diabetes patients have type 2 diabetes mellitus (T2DM), which is evident through mechanisms such as insulin resistance in peripheral tissues, as well as elevated blood glucose levels associated with inadequate insulin secretion10. In T2DM, insulin resistance is the key mechanism in its pathogenesis and it is an important global health issue affecting populations in India (>12.1%), China (>9.7%) and the United States (>8.3%). T2DM carries associated afflictions including cardiovascular diseases, stroke, kidney failure and poor circulation12. These complications affect multiple organs including the eyes, heart, brain, kidneys and skeletal muscle10. A report from the American Diabetes Association states that the number of Americans living with diabetes will increase by 64% by 2025, thus causing a decrease in quality of life and creating consequential social and economic burdens12.
Studies have shown that stem cell therapy may be a useful therapeutic for diabetes mellitus and transplantation of embryonic stem cells have been investigated as potential sources for insulin producing β cell replacement. There is particular potential for stem cells in the skeletal muscle aspects of the disease, where diabetes can lead to various structural, functional and metabolic changes, such as muscle atrophy, muscle weakness and a decrease in energy. Through research it was deduced that transplantation of various stem cells into skeletal muscle may be useful in abating symptoms of diabetes in mice10.
Other studies also portray the safety and therapeutic competence of stem cell therapy in T1DM patients. Findings illustrate that patients attain improved metabolic control and reduced autoimmunity, which lasts for months, following a single treatment of stem cell therapy. Clinical data shows evidence that reversal of autoimmunity leads to regeneration of pancreatic islet β cells and enhanced metabolic control in long-standing T1DM patients11.
With regard to T2DM, evidence points to the involvement of multiple immune dysfunctions in overcoming insulin resistance. Monocytes and macrophages cause chronic inflammation resulting in insulin resistance. Research demonstrates that stem cell therapy can control the immune dysfunctions and restore immune balance through the regulation of monocytes and macrophages, both in peripheral blood and in tissues. This causes an extended reversal of insulin resistance and a significant improvement in insulin sensitivity and metabolic control in T2DM patients12.
Although there is documented success of stem cell therapy in diabetes, studies have also shown that the execution of stem cell therapy in the routine clinical setting is restricted due to a risk of malignancy, autoimmune response and rejection of the transplanted cells9. Research indicates that this problem may be attenuated via use of nanoparticle technology. Cell encapsulation protects therapeutic agents from rejection from the host immune system and overcomes the need for immunosuppression. In a prior study, naked non-encapsulated human embryonic stem cells (SC- β cells) were unable to demonstrate glycaemic correction in immune-competent mice. Conversely, triazole-thiomorpholine dioxide (TMTD) alginate-encapsulated SC- β cells alleviate immune responses by preventing the activation of inherent immune cell populations.
This demonstrated long-term glycaemic correction and glucose responsiveness, without immunosuppressive therapy, in immune-competent diabetic C57BL/6J mice. It is believed that encapsulated human SC- β cells have the capability of providing insulin independence in patients suffering from T1DB13. An additional clinical advantage of SC- β cells, compared with previously proposed therapies using pancreatic precursors, is that these cells do not require an extended post-transplantation period before noticing insulin production. Thus, immediate insulin production makes it feasible to reduce the exogenous insulin requirements of a patient during the first few days post-transplantation14.
Conclusion
Stem cell therapy is widely regarded as the future of medicine. The rapid growth of this mode of treatment has generated excitement in the general population. Its application in self-renewal and differentiation, as well as its relevance in tissue healing and repair, inspires enthusiasm in its feasibility moving forward. Meanwhile, nanotechnology offers exciting new advances in medicine and presents advantages for targeted drug delivery that can overcome biological barriers. Encapsulation allows drugs to be protected from degradation and thus enhances their stability, allowing defined drug/protein delivery and sustained release of the therapeutic agent from the nanoparticle. The compatibility of nanoparticles within biological systems is a key proponent to the treatment and cure of various diseases6,7. Additionally, nanotechnology and encapsulation of therapeutic agents provides a pragmatic platform for controlling stem cell expansion, differentiation and transplantation1.
This review describes the useful function of stem cell therapy and its effective treatment of diabetes mellitus. In addition, an application providing evidence of long-term glycaemic correction and the longest duration of normoglycemia attained via TMTD alginate-encapsulated insulin-producing human embryonic stem cells in a robust rodent model was demonstrated effectively13. Although the application provided is for an animal model chemically induced for type 1 diabetes, investigations for the application of nanotechnology and stem cell therapy in the treatment for type 2 diabetes may be beneficial in providing a long lasting reversal of insulin resistance and an improvement in metabolic control as well as insulin sensitivity in type 2 diabetic patients. Diabetes mellitus is an epidemic creating a significant impact on a global scale. The safety and practical applications of stem cell therapy provides hope and promise for improving treatment and finding a cure. The advantages that stem cell therapy and nanotechnology provide may assist diabetic patients in managing the effects of diabetes and improve quality of life12.
Before any cell-based therapy to treat diabetes makes its way to the clinic, several safety issues must be addressed. A major consideration is whether any precursor or stem-like cells transplanted into the body might revert to a more pluripotent state and induce the formation of tumours or other complications. These risks can possibly be reduced if fully differentiated cells are used in transplantation.
References
- Wilson JL, McDevitt TC. Stem cell microencapsulation for phenotypic control, bioprocessing, and transplantation. Biotechnology and bioengineering. 2013;110(3):667-82
- Lin HT, Otsu M, Nakauchi H. Stem cell therapy: an exercise in patience and prudence. Philosophical transactions of the Royal Society of London Series B, Biological sciences. 2013;368(1609):20110334
- Corradetti B, Ferrari M. Nanotechnology for mesenchymal stem cell therapies. Journal of controlled release: official journal of the Controlled Release Society. 2015
- Lanzoni G, Oikawa T, Wang Y, Cui CB, Carpino G, Cardinale V, et al. Concise review: clinical programs of stem cell therapies for liver and pancreas. Stem cells (Dayton, Ohio). 2013;31(10):2047-60
- Daley GQ. The promise and perils of stem cell therapeutics. Cell stem cell. 2012;10(6):740-9
- Danhier F, Ansorena E, Silva JM, Coco R, Le Breton A, Preat V. PLGA-based nanoparticles: an overview of biomedical applications. Journal of controlled release: official journal of the Controlled Release Society. 2012;161(2):505-22
- Wong IY, Bhatia SN, Toner M. Nanotechnology: emerging tools for biology and medicine. Genes & development. 2013;27(22):2397-408
- Simon-Yarza T, Formiga FR, Tamayo E, Pelacho B, Prosper F, Blanco-Prieto MJ. PEGylated-PLGA microparticles containing VEGF for long term drug delivery. International journal of pharmaceutics. 2013;440(1):13-8
- Berezin AE. Diabetes mellitus and cellular replacement therapy: Expected clinical potential and perspectives. World journal of diabetes. 2014;5(6):777-86
- Fujimaki S, Wakabayashi T, Takemasa T, Asashima M, Kuwabara T. Diabetes and stem cell function. BioMed research international. 2015;2015:592915
- Zhao Y, Jiang Z, Zhao T, Ye M, Hu C, Yin Z, et al. Reversal of type 1 diabetes via islet beta cell regeneration following immune modulation by cord blood-derived multipotent stem cells. BMC medicine. 2012;10:3
- Zhao Y, Jiang Z, Zhao T, Ye M, Hu C, Zhou H, et al. Targeting insulin resistance in type 2 diabetes via immune modulation of cord blood-derived multipotent stem cells (CB-SCs) in stem cell educator therapy: phase I/II clinical trial. BMC medicine. 2013;11:160
- Vegas AJ, Veiseh O, Gurtler M, Millman JR, Pagliuca FW, Bader AR, et al. Long-term glycemic control using polymer-encapsulated human stem cell-derived beta cells in immune-competent mice. Nature medicine. 2016;22(3):306-11
- Pagliuca FW, Millman JR, Gurtler M, Segel M, Van Dervort A, Ryu JH, et al. Generation of functional human pancreatic beta cells in vitro. Cell. 2014;159(2):428-
Biographies
Dr. Yashwant Pathak completed his MS and PhD in Pharmaceutical Technology from Nagpur University, India and EMBA and MS in Conflict Management from Sullivan University, KY, US. He is the Associate Dean for Faculty Affairs at College of Pharmacy, University of South Florida in Tampa, Florida. With extensive experience in academia as well as industry, he has more than 100 publications, two patent applications, five books in nanotechnology and four in nutraceuticals as well as several books in cultural studies. His areas of research include drug delivery systems and its characterisation in animal models. Dr. Pathak’s email address is: [email protected]
Vishal Shahidadpury was born in St. Thomas in the US Virgin Islands and is a current of resident of Sarasota, Florida. Vishal graduated with a Bachelor’s degree in Biology from the University of Florida, as well as a Master’s Degree in Medical Sciences from the University of South Florida. Vishal’s family includes father Haresh, mother Reena, and younger sister Nikita.
Dr. Anjali Hirani received her MS and PhD in Biomedical Engineering from Virginia Tech, Blacksburg, VA. She is currently a postdoctoral fellow at the University of South Florida in the College of Pharmacy. Her research is focused on the development of sustained drug delivery systems for ocular disease.