The limitations of the colony-forming unit in microbiology
Posted: 6 January 2016 | | 1 comment
The recent revision to USP General Informational Chapter <1223> Validation of Alternative Microbiological Methods that became official on December 1, 2015 contained a section discussing the limitations of the colony-forming unit (CFU) in terms of enumerating only those microorganisms that readily grow on solid microbiological media. The section highlights its inappropriateness as a gold standard for method validation when there are many signals available other than CFUs for the detection, enumeration and identification of microorganisms in water, air, pharmaceutical ingredients and drug products.
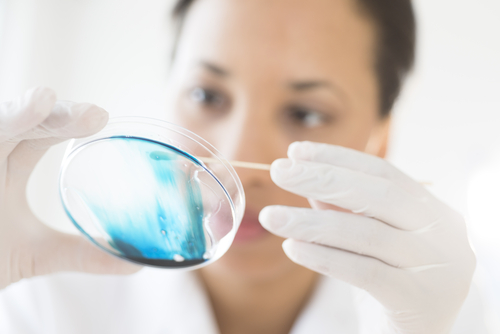
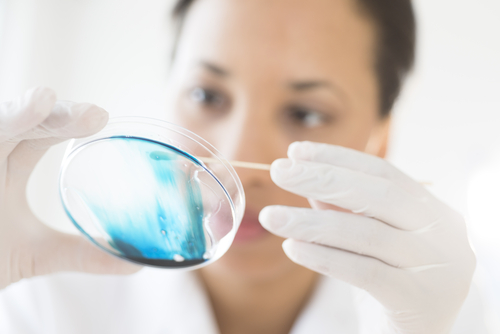
The advent of ribosomal RNA gene sequence analysis thirty years ago has significantly expanded our awareness of microbial diversity in water, air, soil and the human body and reemphasised that, overall, cultivable microorganisms represent less than 1% of the microorganisms observed by direct microscopic examination. This cultivability could range from 0.1 to 0.01% in oceanic microbiota to around 80% for the microbiota on the human forearm. Questions that can be asked include: what does this understanding mean to the pharmaceutical microbiologist responsible for testing and releasing drug products? How does this apply to the challenge of evaluating, validating and implementing alternative methods to the compendial microbiological test methods? As a background to a general discussion of these questions amongst microbiologists, it is useful to review the history of the development of microbiological testing methods.
From Pasteur’s discoveries to the Petri dish
The development of classical microbial methods, broth culture, plate count, serial dilution, enrichment and microbial identification over time has been described in the literature. The method of growing bacteria in a transparent liquid nutrient medium and inoculating fresh medium from turbid medium to isolate a pure culture of the bacterium was commonly employed by the French pioneering microbiologist Louis Pasteur, beginning in 1861. Pasteur used a medium consisting of 100 parts water, 10 parts dextrose, one part ammonium tartrate and one part the ash of yeast cells to grow bacteria. However, before isolation on solid media became a standard practice, it can be said that Pasteur did not truly obtain pure cultures of microorganisms.
The transitional step between liquid and solid culture was the work of Schroeter in 1872, who isolated pigmented bacterial colonies of the now-described red Serratia marcescens and violet Chromobacterium violaceum on thin slices of heat-treated potato. This pioneering work suggested that different bacteria were unique species, since the colour of the pigmented bacteria was consistent when transferred to another potato slice, and inspired the development of solid nutrient media by Robert Koch and his co-workers. To isolate the growth to single points in the medium, the mycologist Julius Brefeld in 1872 added gelatin to liquid media to cultivate moulds from single spores.
To obtain a pure culture Klebs used fractional culture two years later, but this was selective for the microorganism most suitable for growth in the chosen liquid medium. Bacterial enumeration was first achieved in 1878 when the British surgeon Joseph Lister combined serial dilution of soured milk with inoculating media under a coverslip with a dispensing syringe to enumerate lactobacillus.
Working as a country physician, Koch inoculated barnyard mice with diseased tissue from farm animals that died of anthrax, reproducing the disease in these improvised laboratory animals. He cultured the spore-forming bacterium responsible for anthrax in ox aqueous humour, and fixed and stained bacteria with methyl violet on a coverslip for microscopic examination. Later he sealed the preparation in Canada balsam and progressed from illuminating it with direct sunlight to using Abbe condenser and immersion oil, which gave better definition owing to it having the same refractive index as glass. In the summer of 1877, Koch demonstrated to the preeminent German microbiologist Ferdinand Cohn, a Professor of Botany at the University of Breslau, the proof that the specific bacterium Bacillus anthracis caused a specific disease, anthrax. Cohn recognised the importance of the work, helped publish the findings to clearly establish the scientific basis of the germ theory of disease, and helped Koch find positions suitable for a scientist of his enormous talent.
The period that followed the appointment of Koch as the Head of the German Imperial Institute for Infectious Diseases was the most significant five years in the development of microbiological techniques. For the plate technique, Koch essentially took nutrient broth, which supported the growth of pathogens, and added gelatin to make it solid and isolate pure cultures as distinctive colonies on the plate. Two additional steps were needed to give us the familiar solid agar microbiological medium in a Petri dish. Firstly, gelatin, which had the disadvantages of liquefying at above-ambient temperature incubation required by many pathogenic bacteria and was not inert (many bacteria hydrolysed it), was replaced by the gelling agent agar, used in Asian cooking. Secondly, the flat, indented glass plates that supported the solid media were replaced by flat, double-sided 25 x 100mm glass plates that could be sterilised by dry heat, hold the molten nutrient agar poured into it, inoculated and stacked during incubation2.
The progress that Koch and his co-workers had made in the development of microbiological techniques is apparent by the inventory of equipment, including culture vessels, media, disinfectants, water baths, Bunsen burners, inoculating needles, stains, microscopes, and sterilising apparatus that the 1883-4 German Cholera Expedition took to Egypt and India2.
In his book Uncultivated Microorganisms, Microbiologist Salva Epstein7 from Northeastern University reported that Winterburg (1898) recognised the limitations of the CFU early on, observing that the number of cells in his samples did not match the number of colonies growing on a solid microbiological plate. This discrepancy was quantified in 1911 by Amann who found that the non-growing numbers of waterborne bacteria were around 150 times those growing as colonies on an agar plate. Conn8 reported that the total microscopic count of soil bacteria was at least 10 to 100 times greater than plate counts from the same soil samples. He believed that this discrepancy was not due to poor dispersion or the presence of dead cells in the samples but down to the majority of cells not growing on plates.
Plate limitations
More recent studies using fluorescent stains have shown that the total microscopic counts of soil were up to 1000 times that of plate counts9. It has been suggested that plate counts select for rapid growing bacteria and do not favour slow growing bacteria but factors including media selection, gelling agents, elevated temperature during pouring molten agar, incubation conditions and incubation time will influence the recovery on solid media. Similar patterns were observed in marine microbiology. For example, Jannasch and Jones10 in 1958 employed five different cultures and two direct microscopic methods for enumerating bacteria in sea water. Direct microscopic methods on membrane filters showed 10 to 10,000 times as many bacteria than cultural methods including macro-colony counts on nutrient agar, silica gel and membrane filters and micro-colony counts on membrane filters.
The limitations of microbiological culture methods was described by the pioneering American microbiologists, father and son H. W. Conn and H. J. Conn in their text book ‘Bacteriology – A Study of Microorganisms and Their Relation to Human Welfare’. In it, they stated: “Another common application of Koch’s technique is the counting of bacteria. If in the material that is mixed with gelatin or agar every microorganism is separate from every other one and grows as a colony, it is obvious that the number of colonies represents the number of microorganisms in the material plated. This method is commonly used in estimating the number of bacteria in water, milk, soil, or other materials, although to get a small enough number of colonies on the plates to be counted, it is often necessary to dilute the materials. This method is so convenient that it is widely used, but unfortunately many bacteria do not grow on the culture media commonly used, while those that grow often occur in large clumps that do not break up when plated – both of which in fact cause the plate count to be considerably below the actual number of bacteria present in the material”4. Eighty years later, in their influential review article, Staley and Konopka11 coined the catchy term “the great plate count anomaly” to describe this phenomenon that was known to microbiologists for many generations but conveniently often discounted.
As pointed out by Brown and Gilbert in 199512, in most compendial microbial tests, the inoculum are passaged several times on solid media to ensure purity, and harvested by washing the colonies off the plate with suitable diluents. Colony density affects colony size with reduced nutrient availability and the production of inhibitory substances by individual colonies shrinking their overall dimensions. Within a colony, oxygen and nutrient depletion occurs in the core of the colony so that the growth status of individual cells will differ, with the youngest cell at the margins and the oldest at the centre of the colonies. Using bacterial lawns for inoculum preparation or growing cells in liquid culture can largely overcome this heterogeneity.
A genetic revolution
The field of microbial testing progressed still further following Watson and Crick’s discovery of the chemical structure of the DNA molecule in 1955, their Nobel Prize winning publication in Nature marking the beginning of the new field of molecular biology, which would revolutionise both biology and medicine. After their discovery, in 1977, Woese and Fox13 introduced ribosomal RNA base sequence analysis as a measure of microbial phylogenetic diversity and reclassified living organisms into three Kingdoms – Bacteria, Achaea and Eucarya.
Thirty years ago, rRNA analysis was first used to analyse environmental samples from less complex ecosystems like hot springs, copper leaching ponds and deep-sea thermal vents with fewer microorganisms. As reviewed by Amman et al14 in 1995, 5S rRNA molecules were extracted, electrophoretically separated, and sequenced to provide phylogenetic information about the ecosystem. Today, with the introduction of the polymerase chain reaction (PCR), the larger 16S rRNA gene fragment extractions can be rapidly amplified, sequenced and analysed against 16S rRNA databases to identify bacterial species. In 1986, Pace and his co-workers stated that “The simple morphology of most microbes provide few clues for their identification; physiological traits are often ambiguous. The microbial ecologist is particularly impeded by these constraints, since so many microorganisms resist cultivation, which is an essential prelude to characterisation in the laboratory”15. The Woesian revolution was established.
Current perspectives
Despite the concerns of clinical microbiologists Fredericks and Relman16, Koch’s postulates may not be applicable to non-cultivable microorganisms associated with human infection – there is a paucity of novel human pathogens isolated only by culture-independent methods. In contrast to the situation in water and soil microbiology, culture methods have proved more than adequate for clinical microbiology, presumably because pathogens colonise nutrient-rich locations in the body in near pure culture and readily make the transition to laboratory culture. Exceptions may be pathogens that enter a non-cultivable state when exposed to salt water, freshwater or a low temperature outside the human body17.
Limitations of the microbial methods used for water analysis are emphasised in the AWWA/APHA18. As explained in the text, in water microbiology, samples are examined to determine their sanitary quality and the plate count and multiple-tube fermentation methods for the detection and enumeration of indicator organisms are intended to determine the degree of contamination with faecal waste, using coliforms as the indicator. The so-called heterotrophic plate count is determined by pour plate, spread plate or membrane filtration methods with the understanding that it is only an approximation of the number of viable microorganisms in the sample and is useful information about the water quality, judging the efficiency of water treatment processes and the quality of water in a distribution system.
Efforts to improve the recovery of microorganisms using modified culture methods are reported in the literature. New cultivation techniques to improve recovery include altering the medium composition, extending the incubation time, lowering the incubation temperature, and increasing the cell density on solid media to gain an increased diversity of colonies. In the belief that standard microbiological media does not meet the metabolic needs of so-called syntrophic organisms, researchers have added siderphores, vitamins, other carbon sources or essential nutrients. However, as pointed out by Nichols et al19 in 2008, these efforts have not significantly increased the overall microbial recovery rate.
With the adoption of alternative microbiological methods, the development of specifications independent of the CFU may be necessary. With a qualitative limit test such as a sterility test, or in the absence of a specified microorganism test, a statement that the product meets the requirement of the test would be sufficient. For a microbial enumeration test, the measurement would be defined in terms other than CFU, e.g., ATP bioluminescence relative light units, number of genomic units per mL, etc. If these units are not truly equivalent to the CFU, then new specifications based on the alternative signal must be established.
USP <1223> perspective on CFUs and method validation
As alternative microbiological methods move further away from classical methods based on the CFU, the USP needs to respond to this rapid microbiological method validation challenge. For example, the current compendial sterility tests are unsuitable for the emerging short-lived cell-derived drug products, sterile compounded preparations and radiopharmaceuticals, since these methods are growth-based.
Signals other than CFUs that may be used for microbial enumeration and detection include:
- Autofluorescent cells detected by spectrophotometric methods;
- Vital stained cells detected by solid-phase and fluid fluorescent cytometry;
- PCR amplified nucleic acid targeted sequences;
- Number or weight of genomic units;
- ATP levels measured by bioluminescence; and
- Headspace analysis.
These signals may be numerically higher than CFU, since they are not limited by the ability of microorganisms to grow in microbiological media, and may not be directly statistically related. However, I believe that they should move directionally with CFU so as to detect adverse bioburden trends. Cumulative sum (CUM-SUM) charting of the alternative signal will reveal whether changes are occurring over time20. The CUM-SUM score will increase when there is an adverse trend in microbial quality and decrease when there is a favourable trend in the alternative signal that will reflect the microbial population in the samples taken during routine monitoring.
It should be kept in mind that materials with an established fitness for use using CFU will retain their quality level despite the measurement of an alternate signal. The reduced time to a result is most useful with in-process bioburden monitoring where manufacturing can respond to an adverse trend in a timely manner and sterility testing of short-lived products like cell therapies, compounded sterile preparations and radiopharmaceuticals. To take advantage of emerging technologies, we may need to cut the ties to the CFU as a so-called gold standard.
References
- Beck RW. (2000) A chronology of microbiology in historic context. ASM Press
- Block TD. (1988) Robert Koch – A life in medicine and bacteriology. Springer-Verlabg, New York
- Bulloch W. (1938) The history of bacteriology. Oxford University Press, New York
- Conn HW and Conn HJ, (1923) Bacteriology – A study of microorganisms and their relation to human welfare. Williams & Wilkins, Baltimore pp39-54
- Cundell AM. (2003) Historic perspective on methods development In Rapid microbiological methods in the pharmaceutical industry Martin C. Easter, Editor Interpharm/CRC pp9-18
- Handelsman J. Metagenomics: Application of genomics to uncultured microorganisms. Microbiol. Mol. Biol. Rev. 2004; 68(4): 669-685
- Epstein SS. Uncultivated Microorganisms. Springer, New York
- Conn HJ. (1918) The microscopic study of bacteria and fungi in soil. N.Y. Agr. Exp. Sta. Tech. Bull. 2009; 64: 3-10
- Olsen RH and LR Bakken. Viability of Soil Bacteria: Optimization of Plate-Counting Technique and Comparison between Total Counts and Plate Counts within Different Size Groups. Microb. Ecol. 1987;13:59-74
- Jannasch HW and GE Jones. Bacterial populations in seawater as determined by different methods of enumeration. Limnol. Ocanogr. 1959; 4: 128-139
- Staley JT and A Konopka. Measurement of in situ activities of non-photosynthetic microorganisms in aquatic and terrestrial habits. Ann. Rev. Microbiol. 1985; 39: 321-346
- Brown MRW and P Gilbert (1995) Influence of the environment on the properties of vegetative microorganisms: an overview. In Microbiological Quality Assurance – A guide towards relevance and reproducibility of inocula CRC, Boca Raton
- Woese C and G Fox. Phylogenetic structure of the prokaryotic domain: the primary kingdoms. Proc. Natl. Acad. Sci. 1977; 74: 5088-5090
- Amman RI, W Ludwig and K-H Schleifer. Phylogenetic identification and in situ detection of individual microbial cells without cultivation. Microbiol. Rev. 1995; 59 (1): 143-169
- Pace NR, DA Stahl, DJ Lane and GJ Olson. The analysis of natural microbial populations by ribosomal RNA sequences. Adv. Microbiol. Ecol. 1986; 9:1-55
- Fredericks DN and DA Relman. Sequence-based identification of microbial pathogens: a reconsideration of Koch’s postulates Clin, Microbiol. Rev. 1996; 9 (1): 18-33
- Roszak DB and RR Colwell. Survival strategies of bacteria in the natural environment. Microbiol. Rev. 1987; 51(3): 365-379
- AWWA/APHA Standard Methods for the Examination of Water and Wastewater, 20th Edition Section 9010
- Nichols DK, Lewis J, Orjala S, Mo R, Ortenberg P, O’Connor C, Zhao P, Vouros T, Kaeberlein and SS Epstein. Short peptides induces uncultivatable microorganisms to grow in vito. Appl. Environ. Microbiol. 2008; 74(15): 4889-4897
- Bandurek GR. Cumulative sum charts for problem solving. BioPharm Intern. 2008; 21 (5): 1-5
Biography
Dr. Tony Cundell works as a Consulting Microbiologist, supporting the pharmaceutical industry. Prior to November 2013 he worked for Merck Research Laboratories in Summit, New Jersey, as the Senior Principal Scientist in early-phase drug development. Earlier in his career, Dr. Cundell worked at a director level in quality control and product development organisations at the New York Blood Center, Lederle Laboratories, Wyeth Pharmaceuticals and Schering-Plough. He is a member of the 2010-2015 U.S. P Microbiology Committee of Experts. In June 2009, he co-edited with Anthony Fontana a book entitled Water Activity Applications in the Pharmaceutical Industry and contributed two chapters to the book. Tony Cundell has a PhD in Microbiology from the Lincoln University, New Zealand.
Hello, it is important to my daily duties in my pharma job. Thank you