High-throughput PCR based diagnostics: Linking sample handling to molecular oncology risk groups
Posted: 24 June 2010 |
Cancer molecular pathology broadly relies on the comparison between diseased and normal tissues, with statistically validated differences revealing cancerassociated pathways. This approach, although comparatively one-dimensional, has been remarkably successful, enabling identification of many types of malignant biomarkers and providing the means to develop pharmaceutical agents directed against pertinent biological targets. Most typically during the progression of malignancies, pathologists employ morphological screening of cancerous tissues. However, this form of monitoring has significant limitations, particularly in the early stages of pre-treatment or during the clinical remission.
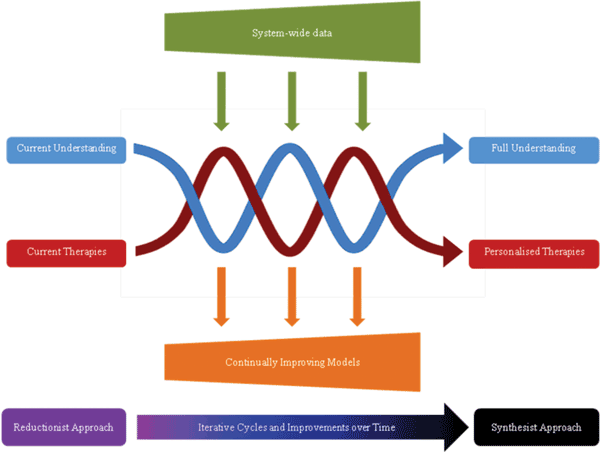
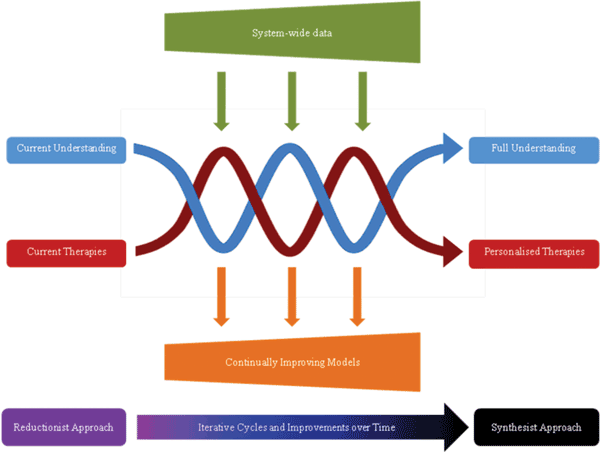
Figure 1 Evolutionary systems biology for personalized medications. Over time, iterative cycles of experimentation and modelling facilitate a move from current understanding and therapeutic options, towards a more complete understanding of disease, with the possibility for personalised therapies. Inputs are needed in the form of system-wide data sets, which are highly anticipated to become increasingly detailed and accurate as analytical technologies improve. Interim benefits are also gained with each advancing cycle, in the form of continually evolving models that improve disease management and guide therapy (Figure produced with Jennifer Logan, University of Liverpool)
Cancer molecular pathology broadly relies on the comparison between diseased and normal tissues, with statistically validated differences revealing cancerassociated pathways. This approach, although comparatively one-dimensional, has been remarkably successful, enabling identification of many types of malignant biomarkers and providing the means to develop pharmaceutical agents directed against pertinent biological targets. Most typically during the progression of malignancies, pathologists employ morphological screening of cancerous tissues. However, this form of monitoring has significant limitations, particularly in the early stages of pre-treatment or during the clinical remission.
Therefore, perhaps not surprisingly, much emphasis has been placed on measurement of cancer-related biomarkers, and particularly for nucleic acids, where analysis to determine pathogenesis is linked to effective therapy. Despite the increasing role given to quantitative PCR (qPCR) in modern diagnostics, the major caveat in its applications is introduced during the stages of sample preparation, an essential step to isolate amplifiable nucleic acids from the population of cells possessing the analyte1. The nucleic acid extraction performance of the different steps of sample preparation, from cell lysis to purification followed by in-vitro gene amplification, can lead to both losses and crosscontamination of analyte. In addition, current sample preparation methodologies are performed on largely heterogeneous clinical cellular matter. Together, the ill-defined sampled mixture of diseased and healthy cells combined with data derived from qPCR bulk measurements contributes to a limited confidence in the measurement of nucleic acids. This compromises the application of qPCR measurements in clinical diagnostics, and particularly for defining disease risk groups. There is a growing urgency to generate quantitative PCR data. This is because the quantification of biomarkers, including nucleic acid measurements, is becoming imperative if personalised medication is to be achieved through the application of systems biology, necessitating holistic mathematical models which are based on the input of quantitative biomarker data.
With perhaps the exception of measurement in biological fluids, future clinical testing might benefit from utilising the cell as a common denominator, and not a unit based on weight or volume. This approach will fit well with the requirements of the systems modellers and will instill standardisation in molecular testing, permitting direct and tighter association of biomarkers with disease and treatment regimes.
Therefore, a next generation of molecular genetics may be required to massively increase PCR throughput, enabling a single sample to be fragmented into sub-reactions, each carrying a single cell. Various platforms are already being developed to facilitate this approach and include digital PCR formats. Quantification of nucleic acids at this level of resolution in diagnostics is novel and has not been attained. Furthermore, the application of this data requires investment in retrospective clinical studies to reveal pertinent biomarkers and use these to define thresholds of disease2.
In spite of its infancy, systems biology tries to bridge this gap by modelling pathway responses to changes in environment for predicting the medical outcome following therapeutic intervention. This is depicted in Figure 1 which relates how a move away from the current reductionist approach to disease analysis and towards a more holistic perspective of disease may permit the realisation of personalised medical healthcare management.
This article is a perspective describing how nucleic acid assay miniaturisation incorpor – ating integrated sample processing with qPCR could connect quantitative pathology to personalised treatment.
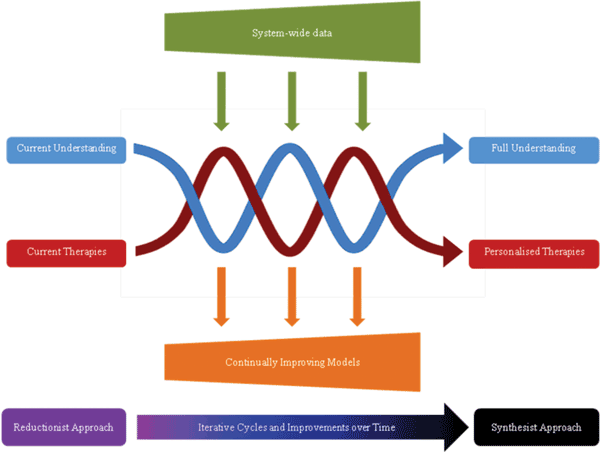
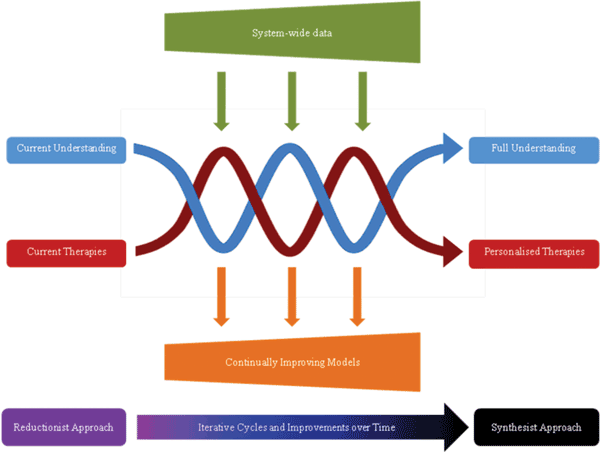
Figure 1 Evolutionary systems biology for personalized medications. Over time, iterative cycles of experimentation and modelling facilitate a move from current understanding and therapeutic options, towards a more complete understanding of disease, with the possibility for personalised therapies. Inputs are needed in the form of system-wide data sets, which are highly anticipated to become increasingly detailed and accurate as analytical technologies improve. Interim benefits are also gained with each advancing cycle, in the form of continually evolving models that improve disease management and guide therapy (Figure produced with Jennifer Logan, University of Liverpool)
Sample preparation
The qPCR process has been highly honed and recently, standardisation of this analytical procedure has been introduced through implementation of Minimal Information about a qPCR Experiment (MIQE)3. Nucleic acid extraction long predates PCR, and as yet does not benefit from standardisation. For the introduction of personalised medication, and to improve on current analyses such as minimal residual disease testing, standard operating procedures that encompass all manipulations from initial sample extraction through to eventual data analysis has proven difficult to routinely accomplish between typical laboratories. From our perspective, only a unit describing biomarker molecules per cell will enable assessment of cellular activity and the definition of disease thresholds. Such an eventuality will also fit well with the integration of biomarkers to permit understanding of normal and disease processes via systems modelling. The miniaturisation of nucleic acid and other bio – molecular analyses, utilising lab on a chip, or micro total analytical systems (μTAS) offers the most promising route to a comprehensive analysis.
Use of miniaturisation for the PCR quantification of disease-associated nucleic acids
Extending the use of microfluidics by μTAS further enables sample tissues to be analysed by employing homogeneous extraction methodologies, whereby no extractions are performed, only reagents are added to nucleic acid solutions, and therefore sample losses will be eliminated by avoiding conventional extractions4,5. The aim of μTAS qPCR is to manoeuvre clinical tissue samples within microchannels and across miniature structures (Figure 2) to improved quantitative analysis of informative target nucleic acid sequences1,6. The major advantage offered by μTAS over more usual instrumentation is that the process of sample treatment and analysis can be tightly regulated1 and adapted for different sample types. For example, with the development of μTAS, target gene identification of malignant cells within peripheral blood can be modified for the analysis of bone marrow and even solid tumours.
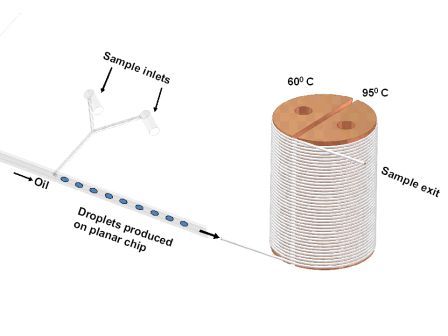
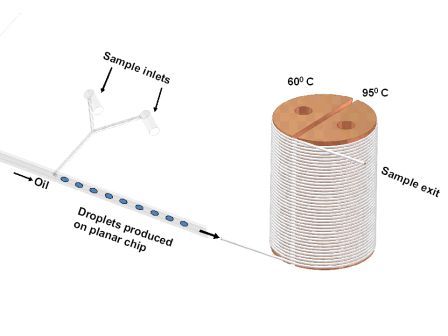
Figure 2 How microfluidic instrumentation applied to handle soft tissue samples, lyse, perform PCR and finally analysis
Dropletised μTAS
Dropletisation for quantification of nucleic acid assays has recently transformed the way in which DNA sequencing is performed. The concept of digital PCR to gain information through dilution of a sample to better defined molecular numbers or even achieve single cell or single molecule targets has been taken a stage further with a group of techniques often termed as next generation sequencing. The formation of randomly generated droplets or emulsions (emulsion PCR) is facilitating new means to rapidly sequence organisms and resequence informative genome segments. The production of droplets on demand in microchannels, which comprise a stream of aqueous droplets separated from each other by a second immiscible oil phase, permits the precise placement in a microchannel (in space) of biological matter to form a vessel in which PCR is performed. One of these miniaturised structures permits a droplet to be made through the fusion of sample together with PCR reagents. The PCR is contained within a droplet and segregated by oil from other droplets to prevent sample losses and cross-contamination. Because the droplets are produced in a moving stream within the microchannel, several thousands of PCRs are possible per hour, per microchannel.
Droplet formation is optimised by altering the flow rates of the aqueous carrier fluid and the oil phase. However, alterations to the flow rates of two phases also impacts on the rate of droplet formation, the dimension of droplets generated, the time each droplet dwells within a PCR cycle, and finally the experimental throughput7.
Quantification of nucleic acids and its future
In general terms, there are two categories of clinical samples which are either liquids or solids. For the majority of the genetic-based analysis of clinical samples, the important constituent within all tissues is the cell. Thus, solid tissues are often allied with a three-dimensional arrangement where cell–cell proximity has significance, and of course, this feature is missing from biomedical fluids. Using μTAS with channel dimensions resembling cell diameters (often 6 – 20 μm in diameter), enables cells in droplets to be analysed individually2. Data generation comprising a group of single cells that is representative of the whole tissue population is a novel approach to disease analysis. However, sampling huge numbers of cells may be impractical, and indeed the analysis of defined cell clusters, such as with laser micro-dissection, may be similarly informative in cases where the genetic biomarker remains discernible.
In the specific case of microdroplet-based PCR, the order of the cells (in droplets) entering the microchannel remains unaltered throughout the analytical process. Therefore, if cells are removed from a solid tissue (employing a means of recording their original location) and placed into the flowing two phase qPCR system, the tissue can in effect be rebuilt in virtual space in accordance with the original 3D location of the cells, and displaying the nucleic acid content of each measured cell.
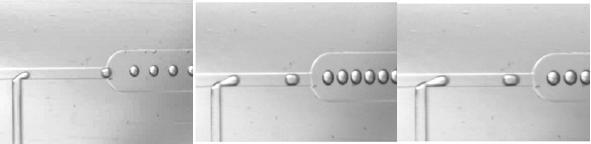
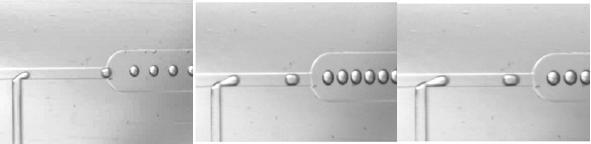
Figure 3. The aqueous droplets containing cells are formed in this platform through the shear force generated at the T-junction where the inlet carrying the aqueous phase meets the oil flow.
This approach to quantitative sample analysis is presenting new and intriguing challenges that relate to the sensitivity and use of quantitative output data from these newly evolving instruments. For example, with Philadelphia-positive leukaemia, there are different numbers of the BCR-ABL fusion transcripts present per cell, and the significance of this is not fully characterised. The evolving field of minimal residual disease is progressively providing higher sensitivity of detection limits. However, these limits of detection are based around technical limits that may not be defining biologically meaningful thresholds. A new generation of qPCR-based instrumentation delivering copy numbers of nucleic acids per disease-associated cell will help to decipher the relevance of disease heterogeneity within an individual and across disease patient cohorts.
A fascinating aspect of the development of microfluidics for application in qPCR is how a relatively modest alteration in sample handling and integration into the PCR process could well transform medicine. This is a bold statement, and is finding increasing popularity. PCR has transformed nucleic acid analysis by providing unrivalled potential for detection sensitivity. Provision of upstream sample handling appropriate to molecules per cell resolution is hoped to make ubiquitous quantitative nucleic acid measurement routine when linked to qPCR. For the present, compartmentalisation of PCR via emulsions, droplets, dilution or other means is to some extent routinely available. With technical limits of detection removed, quantitative nucleic acid data is amenable to help build mathematical models of disease, but its general impact on disease management and treatment is less clear to predict. A great many current diagnostic tests and treatments are already adequate for successful disease management, however there is no reason to resist further improvements to pre-existing testing which seem appropriately poised to deliver personalised medications in the future.
References
1. Chen, L., Manz, A. and Day, PJR. Whole genome amplification on poly(dimethylsiloxane) microchip array. Anal. Biochem. 372, 128–130. (2008).
2. Day, PJR. Miniaturization applied to analysis of nucleic acids in heterogeneous samples. Expert Rev. Mol. Diagn. 6, 23–28. (2006).
3. Bustin SA, Benes V, Garson JA, Hellemans J, Huggett J, Kubista M, Mueller R, Nolan T, Pfaffl MW, Shipley GL, Vandesompele J, Wittwer CT. The MIQE guidelines: minimum information for publication of quantitative real-time PCR experiments. Clin Chem. Apr;55(4):611-22 (2009).
4. Mohr S, Zhang YH, Macaskill A, Day PJR, Barber RW, Goddard NJ, Emerson DR, Fielden PR. Numerical and experimental study of a droplet-based PCR chip. Microfluid. Nanofluid. 3 611–621. (2007).
5. Kiss MM, Ortoleva-Donnelly L, Beer NR, Warner J, Bailey CG, Colston BW, Rothberg JM, Link DR, Leamon JH. High-throughput quantitative polymerase chain reaction in picoliter droplets. Anal. Chem. (2008).
6. Chen, L., West, J., Auroux, P.-A., Manz, A. and Day, PJR. Ultrasensitive PCR and real-time detection from human genomic samples using a bi-directional flow reactor. Anal. Chem. 79, 9185–9190. (2007).
7. Markey AL, Mohr S, Day PJR, High-throughput droplet PCR. The ongoing Evolution of qPCR, edited by Michael W. Pfaffl. Methods, 50, Issue. 4. 2010.
About the Authors
Stephan Mohr
Stephan Mohr is Researcher at the University of Manchester specialised in microfluidics and its applications in biology, chemistry and medical research. He received his degree and PhD in Physics in Goettingen, Germany.
Ehsan G. Karimiani
Ehsan G. Karimiani is a medical doctor, recently qualified with an MRes in translational medicine and is currently studying for a PhD in quantitative genomics at the University of Manchester. His PhD project aims to measure and analyse key transcripts within populations of single leukemic cells at high resolution by employing miniaturised qPCR micro total analysis system (μ-TAS).
Philip Day
Philip Day is Reader in Quantitative Analytical Genomics, Manchester University, Director of Quantitative Molecular Medicine and Principal Investigator at the Manchester Interdisciplinary Biocentre. He develops innovative tools for cancer studies, including miniaturisation for single cell analyses, and works closely with groups in analytical instrumentation, miniaturisation, data analysis, text mining and systems biology. He is an examiner for several research councils and journals, and a member of the Editorial Board of Integrative Biology.
Contact the Author
email: [email protected]