The scope of PAT in real-time advanced control of tablet quality
Posted: 20 April 2015 |
Continuous pharmaceutical manufacturing together with process analytical technology (PAT) provides a suitable platform for automatic feed-forward/feed-back (FF/FB) control of the end product quality as desired by quality by design (QbD)-based efficient manufacturing. The precise control of the quality of the pharmaceutical product requires proactive, corrective actions on the process/raw material variability. Therefore, PAT tools are necessary to monitor the FF as well as FB process variables that need to be sent to the automatic real-time control system. This article highlights the scope of PAT in a combined FF/FB control system of a continuous tablet manufacturing process.
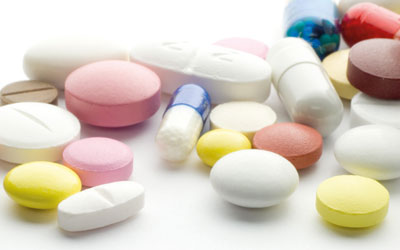
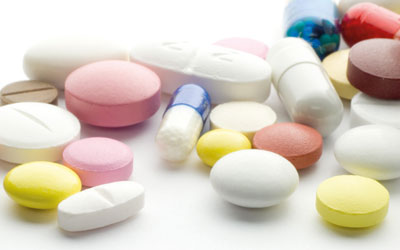
Extensive development on PAT in recent years provides a suitable platform for a paradigm shift of QbD-based pharmaceutical manufacturing. Today, commercially-available methods and tools make the application of PAT possible for real-time pharmaceutical process control, to enable real-time product release within a highly regulated environment. Moreover, recently developed continuous pharmaceutical manufacturing techniques involving solid dosage forms catalytically accelerate this revolutionary shift by enabling both FF and FB control strategies.
The FF controller takes into account the effect of process disturbances and raw material variability proactively while the FB control system ensures consistency in end product quality. For FF control, the sensor (e.g., near infrared; NIR) should be placed upstream to measure the process disturbances and the measured signal should be transmitted in real time to downstream unit operation to take the compensative actions. Variations in raw material properties (e.g., particle size), feeder hopper level, amount of lubrication and milling and blending action, applied shear in different processing stages, can affect the blend density significantly and thereby also affect tablet weight, dissolution and hardness. Therefore, the inline real-time monitoring of the blend density and its incorporation into the control system so that it does not affect the end product quality is highly desired. For FB control, the sensor should be placed at downstream unit operations to measure the critical quality attributes (CQAs) and critical process parameters (CPPs) and based on that the process parameters need to be manipulated to control the measured variables.
In the past few years, very few attempts have been made to control a tablet manufacturing process by utilising a FB control algorithm along with PAT tools4-5. But when such methods are used, it is important to note that FF control systems need to be coupled with the FB control system for it to work effectively. The coupled FF/FB control system ensures minimum variability in the final product quality irrespective of process and raw material variations and it is very successful in different manufacturing industries. The FF control loop is based on real-time monitoring of the powder bulk density while the FB control loops are based on the drug concentration, powder level, tablet weight and hardness. We propose an NIR-based real-time monitoring of the blend density for FF control.
The process
A continuous direct compaction tablet manufacturing pilot-plant has been installed and situated at C-SOPS, Rutgers University5. The process flowsheet model is shown in Figure 1. To describe the set-up, there are three gravimetric feeders, a co-mill, a continuous blender and a tablet press. The NIR sensor for inline monitoring of powder blend uniformity, powder blend composition and powder blend density has been integrated through a chute placed in between tablet press and blender. The local level controllers are inbuilt in each feeder in order to control the powder flow rate. A ratio controller has been added that provides the flow rate set points of API, Excipient and Lubricant feeders for a given total flow rate and API composition. Six supervisory control loops have been then added.
The first loop is for PAT based FF control (FFC) which takes the corrective action on variations in powder bulk density. Powder blend is fed to the tablet press die through a feed frame. For a specific fill depth and punch displacement settings, the variation in blend density can lead to variations in the tablet weight, hardness and dissolution. The powder blend density during continuous tablet manufacturing operation can change at different processing units for several reasons. For example, the powder hopper level can change the powder bulk density. Powder particle size has significant effects on the powder bulk density. So if the raw material specification has been changed, then it can have significant effects on the final product quality if a suitable control strategy has not been implemented. The shear level can also change the powder bulk density. Furthermore, the powder bulk density can alter during feeding operation because of shear introduced by helix. Milling operation can also change the powder bulk density because of change in particle size and applied shear force. The blending operation changes the bulk density because of shear force. The bulk density can also change during the powder flow for several reasons; for example segregation and compression. An NIR sensor together with chemometric tools has been used for real-time inline monitoring of the powder blend density. The signal of powder blend density is then sent to the feed-forward controller that manipulates the fill cam depth of the tablet press proactively.
The second loop has been added to control the main compression force of the tablet press. This control loop is in cascade arrangement with a master controller (loop 3) specifically designed to control the tablet weight. The input of this master controller is weight and it generates the set point of main compression force. Loop four has been designed to control the tablet hardness by manipulating the punch displacement. Checkmaster (Fette) has been used for real-time monitoring of tablet weight and hardness. Loop 5 has been added to control the powder level in instrumented hopper and a webcam has been used for online monitoring of the powder level. The drug concentration is controlled through the PAT-based 6th control loop, while the NIR sensor has been used for inline real-time monitoring of drug concentration. The powder bulk density and drug concentration can be monitored using a single NIR probe. Two PLS models have been used to predict the powder bulk density and drug concentration separately.
In order to implement the designed FF/FB control into the pilot-plant, the signal from the NIR sensor (raw spectrums) has to be sent to a chemometric tool that utilises the NIR calibration models for blend density and drug concentration and a real-time prediction tool generates the signals for the control variables in real time. The generated signals are then sent to a commercially-available control platform via an OPC (OLE process control) where the combined FF/FB control loop has been implemented. The blend density is the input for the FF controller while the blend composition together with powder level, tablet weight and hardness are the inputs of the FB control system.
Real-time measurement of powder bulk density, drug concentration and blend uniformity
NIR has in the past been used for the real-time monitoring of powder bulk density, drug concentration and blend uniformity, the latter two of which have been previously reported4-6. Here, we describe real-time monitoring of powder bulk density using an NIR sensor. The first step in the process is to calibrate the NIR. In order to develop the NIR calibration model, the spectrums need to be collected for powder samples of different densities. The powder has been filled in a graduated cylinder placed on the top of a tapping machine. Then the taps have been applied on the graduated cylinder.
Tapping changes the bulk density of the powder and thereby the powder volume. Based on the change in the volume, the reference values of the density can be calculated. The highest changes in density are obtained for the first 20 taps. The spectrums have been collected for each density. The PLS-based calibration model for density is developed using a chemometric tool and an NIR sensor is integrated with the direct compaction tablet manufacturing pilot-plant through a chute interface. The calibration models for density and drug concentration are then integrated with a chamometric tool for real-time prediction of density and concentration signals, respectively. Blend uniformity (relative standard deviation) can then be calculated from the drug concentration signals.
A proprietary PAT data management tool (synTQ) is used for the management of raw data and predicted signals. The data across different software tools communicate via OPC interface. In gravimetric mode of the operation of lose-in-weight feeder, the powder flow rate is controlled by manipulating the feeder screw speed. So if there is variation in the powder bulk density then the screw speed needs to be adjusted automatically in order to deliver the same mass flow rate of the powder. Therefore, the feeder screw speed is the primary indication of the change in the powder bulk density.
Results and discussion
The real-time monitoring of powder bulk density along with feeder screw speed is shown in Figure 2. As shown in the figure, the screw rotational speed first decreases and then increases indicating that the powder density slightly rises before dropping non-linearly. The figure demonstrates how the change in bulk density affects the feeder screw rotational speed. The density measurement response is delayed in comparison to feeder screw speed response. This result demonstrates the proof of concept that NIR can be used for real-time monitoring of powder bulk density.
The effect of powder bulk density on tablet weight is shown in Figure 3. Step change in the powder bulk density has been introduced by switching the excipient from Avicel 101 to Avicel 301 and back to Avicel 301. Avicel 301 is known to be denser than Avicel 101. API (APAP) and lubricant (MgSt) remains same.
Figure 3 shows that powder bulk density has a significant impact on tablet weight. Similar effects have been observed on tablet hardness as well. Therefore, the powder bulk density is critical to monitor for real–time feed-forward control. It can be proposed that the effect of density variation is compensated by adjustment of the fill depth. Meanwhile, Figure 4 shows the sensitivity of fill depth on tablet weight and hardness. The results demonstrate that the fill depth can be manipulated to compensate the variation in density so that a consistent tablet weight and hardness can be achieved.
Conclusion
This review highlights the scope of PAT for FF/FB control to obtain a precise pre-defined end-product quality of a pharmaceutical product, as mandated by regulatory authorities. NIR has been used for real-time monitoring of powder bulk density, drug concentration and blend uniformity. Future work includes the full implementation of an FF/FB control system into our pilot-plant.
Acknowledgements
This work is supported by the National Science Foundation Engineering Research Center on Structured Organic Particulate Systems, through Grant NSF-ECC 0540855.
References
- (2004). PAT—A framework for innovative pharmaceutical development, manufacturing, and quality assurance. http://www.fda.gov/downloads/Drugs/Guidances/ucm070305.pdf
- (2007). Guidance for industry, Q8 (R2) pharmaceutical development. http://www.fda.gov/downloads/Drugs/Guidances/ucm073507.pdf
- García-Munoz , S. Dolph, H. W. Ward II. Handling uncertainty in the establishment of a design space for the manufacture of a pharmaceutical product. Computers and Chemical Engineering 2010, 34, 1098-1107.
- Singh, R.; Sahay, A.; Karry, K. M.; Muzzio, F.; Ierapetritou, M.; Ramachandran, R. Implementation of a hybrid MPC-PID control strategy using PAT tools into a direct compaction continuous pharmaceutical tablet manufacturing pilot-plant. International Journal of Pharmaceutics 2014, 473, 38–54.
- Singh, R.; Sahay, A.; Muzzio, F.; Ierapetritou, M.; Ramachandran, R. Systematic framework for onsite design and implementation of the control system in continuous tablet manufacturing process. Computers & Chemical Engineering Journal 2014, 66, 186-200.
- Vanarase, A.; Alcal, M.; Rozo, J.; Muzzio, F.; Romaach, R. Real-time monitoring of drug concentration in a continuous powder mixing process using NIR spectroscopy. Chem. Eng. Sci. 2010, 65 (21), 5728 – 5733.
Biographies
Dr. Ravendra Singh is Assistant Research Professor at Department of Chemical and Biochemical Engineering, Rutgers University, USA. He is the recipient of a prestigious EFCE Excellence Award given in Recognition of an Outstanding PhD Thesis, from European Federation of Chemical Engineering. He is a recognised scientist in the field of continuous pharmaceutical manufacturing, PAT and process control, and has published 40 research articles in international journals and magazines, written two book chapters, has given several invited industrial lectures and presented at over 50 international conferences. He is lead guest editor of Journal of Chemistry special issue for PAT.
Dr. Marianthi Ierapetritou is a Professor and Department chair of Chemical and Biochemical Engineering at Rutgers University in Piscataway, New Jersey. She obtained her BS from National Technical University in Athens, Greece (magma cum laude), her PhD from Imperial College (London, UK) in 1995 and subsequently completed post-doctoral research at Princeton University (Princeton, NJ) before joining Rutgers University in 1998. Among her accomplishments are the Outstanding Faculty Award in 2012, the Rutgers Board of Trustees Research Fellowship for Scholarly Excellence in 2004, and the prestigious NSF CAREER award in 2000.
Dr. Rohit Ramachandran is currently an Assistant Professor at the Department of Chemical & Biochemical Engineering, Rutgers University, New Jersey, USA. He completed his bachelors and masters in Chemical Engineering at the National University of Singapore. This was followed by his PhD in Chemical Engineering at the Centre of Process Systems Engineering, Imperial College London. He then undertook postdoctoral work at MIT in the Process Systems Engineering Laboratory and the Novartis-MIT Center for Continuous Manufacturing. His research interests include modeling, control and optimisation of pharmaceutical and chemical processes.
Issue
Related topics
Formulation, Process Analytical Technologies (PAT), Quality by Design (QbD)