Hydrogel encapsulation: unlocking the potential of cell therapies for targeted treatments
Posted: 7 January 2025 | Francis Karanu (Likarda), Stephen Harrington (Likarda) | No comments yet
In this article, Dr Stephen Harrington, Director of Cell and Hydrogel Formulation and Francis Karanu, Vice President of Cell Therapy R&D, Likarda, discuss the benefits of hydrogel encapsulation in drug delivery and enhancing the bioavailability and functionality of cell therapies.
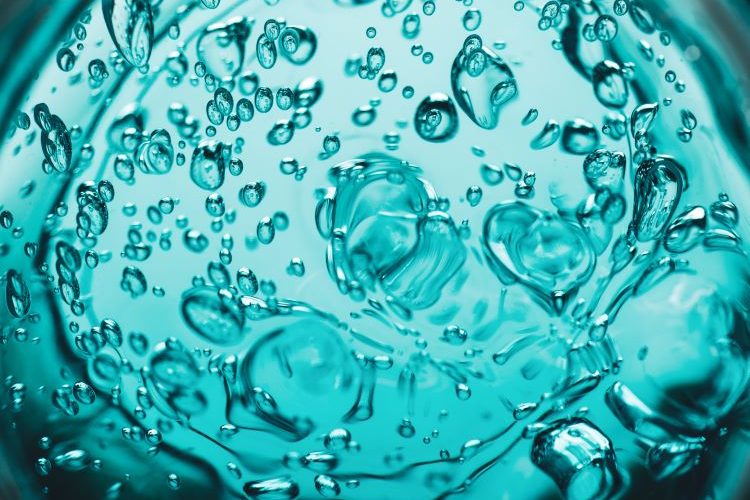
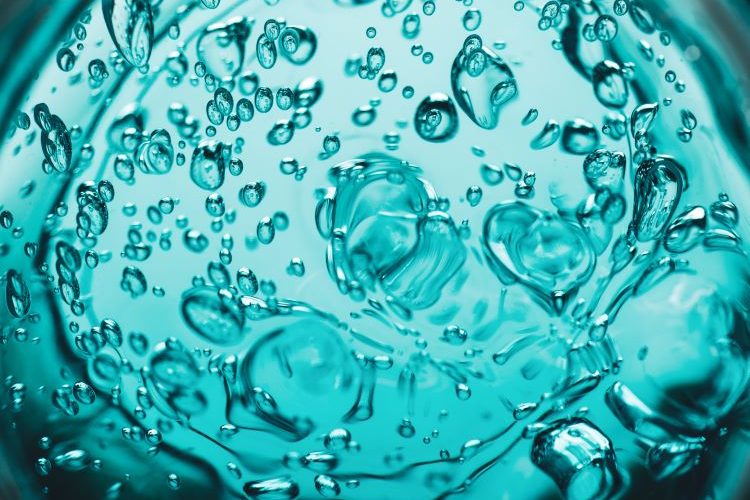
With an explosion of new cell therapies in the pipeline, a key challenge in the field remains getting these treatments from the lab and into the patient so that they continue to function in vivo.
Cell encapsulation is a promising strategy for addressing this issue. The use of hydrogels as excipients for the delivery of many therapeutic agents has become increasingly relevant, owing to their many advantages.1 Cells can be delivered to a specific location in a scaffold that protects them from immune attack while allowing diffusion of cytokines out of the scaffold, also enabling the slow release of the cells into the region.
While the concept is simple, the encapsulant must have specific qualities that enable the cells to thrive while not eliciting an inflammatory or immune response.2 It must be injectable into small areas such as joints, should not induce pain, and its breakdown components must be safe.3
The theoretical benefits of using hydrogel formulations to deliver cell therapies are varied, based on the product in question, and revolve around six aspects: immunological tolerance, biocompatibility, bioavailability and targeted delivery, stability and maintenance of cell function.
Immunological tolerance
By providing [a physical] barrier and localised immune effects, hydrogels can provide critical benefits in cell therapy procedures that include prolonged cell survival and hence lead to greatly improved patient outcomes.6″
Hydrogel formulations play key roles in immunological tolerance by protecting cells from immune rejection and providing an enabling environment for cell survival, proliferation, migration and functionality. Hydrogels achieve this by supplying a physical barrier that separates the immune system – including T cell, B cell and macrophages – from the therapeutic cells, while allowing diffusion of oxygen and nutrients and removal of waste products.4,5 The effectiveness of hydrogels in providing immune tolerance can be further enhanced by the use of local immunomodulator agents, including cytokines and other agents that provide localised effects, as opposed to systemic effects which may not always be desirable.
By providing this barrier and localised immune effects, hydrogels can provide critical benefits in cell therapy procedures that include prolonged cell survival and hence lead to greatly improved patient outcomes.6
Biocompatibility
Hydrogels offer additional extracellular-like matrices that enhance the adhesion, proliferation, migration and viability of cells. Beyond biocompatibility with the encapsulated cells, it is equally important that the hydrogel is biocompatible with the surrounding tissues.3″
Hydrogels offer additional extracellular-like matrices that enhance the adhesion, proliferation, migration and viability of cells. Beyond biocompatibility with the encapsulated cells, it is equally important that the hydrogel is biocompatible with the surrounding tissues.3
In fact, failed clinical trials have demonstrated what a non-biocompatible hydrogel can do. In one clinical trial, a non-biocompatible alginate hydrogel was used to encapsulate cells to treat haemophilia A. After administration, the patients had a severe reaction, necessitating surgical removal of the microspheres, which subsequently revealed significant fibrosis on the microbeads’ surface caused by an immune response to the alginate.7 Thus, it is essential to ensure biocompatibility of the hydrogel material.
Bioavailability and targeted delivery
Hydrogels significantly enhance the bioavailability of therapeutic agents that are often delivered locally to act in specific desired sites,3 or by acting systemically through release of materials that are taken up and circulated by the blood system.5,6 These gels offer protective, supportive and regulated environments that enhance cell survival and functionality.
When considering bioavailability in the traditional sense, therapeutic cells are often administered systemically by injection into the vasculature such as when haemopoietic cells are injected into the blood stream, where they naturally home to bone marrow.
However, it is now understood that outside of haemopoietic cells that target blood cancers, therapeutic cells do not home to a location. Thus, local delivery of cells to a target site can improve bioavailability, but without a delivery material or genetic modifications, cells do not stay where they are injected.3 Thus, hydrogels used in local cell delivery offer improved bioavailability.8
New technologies like hydrogel microbeads are used as a local delivery system both to deliver the molecules that cells secrete, such as insulin and albumin, as well as deliver the cells themselves.3 There are certain conditions that necessitate physical interaction of the therapeutic cells with the cells in a recipient’s surrounding tissue. For these situations, a hydrogel formulation that slowly releases the cells into the localised area can be preferable.3,9
Stability
hydrogels are becoming increasingly important in the cryopreservation, storage and transportation of cell therapies”
If we consider hydrogels used for cell delivery analogous to the inactive ingredients in traditional drugs, we would expect them to both increase the efficacy of the active ingredient and potentially help with storage and shipping issues.
As such, hydrogels are becoming increasingly important in the cryopreservation, storage and transportation of cell therapies. In recent efforts, hydrogels have helped in cell cryopreservation by providing a sustainable environment for cells and impeding ice crystal formation, thus preventing mechanical injury during the freeze and thaw processes. There are several other benefits of using hydrogels in cryopreservation and cell storage, including enabling reduced amounts of toxic cryoprotectants like dimethyl sulfoxide (DMSO), controlling osmotic shock and other cell damaging effects of cryopreservation, and extending the health of cells held at warmer temperatures such as room temperature or 4°C.
Functionality
The overall functionality of cells used as therapeutics determines effectiveness, duration of action and required doses needed to achieve the desired outcomes. Thus, in addition to the delivery of viable cells to the right location, the function of the cells is a key parameter that influences the therapy’s success.3
Hydrogels have the ability to enhance cell function by many of the processes already discussed, including the supportive, biomimetic and controlled environment that is critical to cell survival proliferation, migration and function.9,10
While cells often function well in cell culture, they lose their function after being implanted in the body. In such cases, the hydrogels can be impregnated with survival factors and other signalling molecules that provide additional benefits including maintaining the desired function of the cells.
Occasionally, cells work best when they are near other cell types. For example, pancreatic endocrine cells are supported by nearby exocrine cells. In other situations, cell therapies may require local anti-inflammatory cells to fully elicit their effect. Within a hydrogel, different cell types and essential factors can be co-localised into a more supportive environment for the therapy to work correctly.
Summary
While hydrogels have limitations, there is great promise in the safe microencapsulation of cells using slow-hardening biocompatible hydrogels that impart little or no foreign body response, while creating supportive environments for the therapeutic cells.2 These technologies represent a growing opportunity to better translate the outcomes seen in preclinical testing to dramatically improve patient health in multiple areas with high unmet need.
EPR’s Issue 5 2024 – Read the full issue…
About the authors
Stephen Harrington, PhD, is Director of Cell and Hydrogel Formulation, Likarda. In graduate school at the University of Kansas, Stephen began investigating the application of biomaterials for improving islet transplantation, leading to the development of a novel micro-encapsulation process which was granted a US Patent in 2017, due to its significant potential in cell-based therapy as an injectable, immunoprotective and customisable delivery system.
His research with Likarda also led to several advancements in islet isolation and cryopreservation techniques, and he gained recognition as a 2017 Argersinger Dissertation Award Nominee for Outstanding Doctoral Dissertation at the University of Kansas.
Francis Karanu, PhD, is Vice President of Cell Therapy R&D, Likarda. Prior to joining Likarda, Francis worked at Johnson & Johnson in a stem cell and regenerative medicine venture developing beta-like, insulin-producing cells from pluripotent stem cells for treating diabetes in humans. Francis’ methods for purifying cells derived from pluripotent stem cells were granted a US patent. He has been author or co-author of many peer-reviewed publications and patents. Francis has a PhD in immunology and cell biology from Washington State University, US.
References
1. Li L, Duan X, Fan Z, et al. Mesenchymal Stem Cells in Combination with Hyaluronic Acid for Articular Cartilage Defects. Scientific Reports. 2018; 8(1).
2. Harrington S, Ott L, Karanu F, et al. A Versatile Microencapsulation Platform for Hyaluronic Acid and Polyethylene Glycol. Tissue Engineering Part A. 2020; 27(3-4).
3. Hamilton M, Wang J, Dhar P, Stehno-Bittel L. Controlled-Release Hydrogel Microspheres to Deliver Multipotent Stem Cells for Treatment of Knee Osteoarthritis. Bioengineering. 2023; 10(11):1315.
4. Wang Y, Huang R, Lu Y, et al. Immuno-Protective Vesicle-Crosslinked Hydrogel For Allogenic Transplantation. Nature Communications. 2024; 15(1).
5. Harrington S, Williams J, Rawal S, et al. Hyaluronic Acid/Collagen Hydrogel as an Alternative to Alginate for Long-Term Immunoprotected Islet Transplantation. Tissue Engineering Part A. 2017; 23(19-20):1088–99.
6. Harrington S, Karanu F, Ramachandran K, et al. PEGDA Microencapsulated Allogeneic Islets Reverse Canine Diabetes Without Immunosuppression. PLOS ONE. 2022; 17(5).
7. Sigilon Reports Trial Updates for Investigational Hemophilia A Therapy. [Internet] National Bleeding Disorders Foundation. 2021. [Cited 2024Oct]. Available from: https://www.bleeding.org/news/sigilon-reports-trial-updates-for-investigational-hemophilia-a-therapy
8. Kaleka CC, Zucconi E, Vieira S, et al. Evaluation Of Different Commercial Hyaluronic Acids As A Vehicle For Injection Of Human Adipose-Derived Mesenchymal Stem Cells. Revista Brasileira de Ortopedia (English Edition). 2018; 53(5):557–63.
9. Hamilton M, Harrington S, Dhar P, Stehno-Bittel L. Hyaluronic Acid Hydrogel Microspheres for Slow Release Stem Cell Delivery. ACS Biomaterials Science & Engineering. 2021; 7(8):3754–63.
10. Solis MA, Chen YH, Wong TY, et al. Hyaluronan Regulates Cell Behavior: A Potential Niche Matrix for Stem Cells. Biochemistry Research International. 2012; 2012:1–11.
Issue
Related topics
Biologics, Drug Delivery Systems, Formulation, Industry Insight, Technology