Using rack systems to reduce thermal interactions between vials during freezing
Posted: 11 July 2024 | Fiora Artusio (Politecnico di Torino), Roberto Pisano (Polytechnic of Turin), Vincenzo Massotti (Politecnico di Torino) | No comments yet
Formulation experts from Polytechnic of Turin, Italy, discuss the impact of freeze-drying on the integrity and stability of pharmaceuticals and how mathematical modelling can improve understanding of the freezing process.
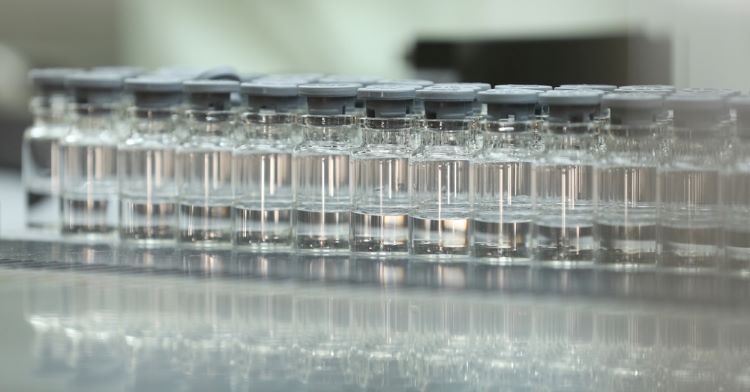
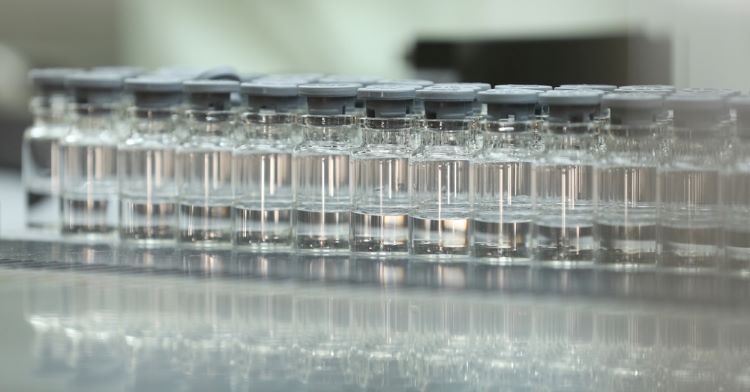
The distribution of pharmaceuticals in vials often requires freeze-drying to preserve drug stability. Vials can be frozen on a temperature-controlled shelf or nested in a rack system. The loading configuration influences the thermal profiles experienced by the product during freezing. For example, nests reduce heat transfer from/to the shelf and mitigate thermal interactions among vials during freezing. Mathematical modelling can help understand the impact of the loading configuration on the distribution of nucleation temperatures and freezing times.
Freezing is a crucial step of pharmaceutical manufacturing as it enhances their shelf-life. However, it may also promote drug denaturation because of the formation of the ice-water interface and/or lead to heterogeneity within the lot due to thermal gradients within the batch of vials or even within the same vial. The thermal history experienced by the pharmaceutical product during freezing is impacted by a complex set of parameters, including freezing conditions, loading configuration of the vials, and packing arrangements.
In the case of shelf-freezing, vials can be loaded directly on the shelf or nested in suitable rack systems. Most commonly, vials are loaded on the shelf with the help of a tray. Recently, racking devices for vials have been developed not only to deliver glass vials from the manufacturer to the pharmaceutical companies, but also as a loading device to be used in the freezing/freeze-drying process.1,2 Vials are therefore nested in their secondary packaging, which prevents vials breakage during transportation, and are used as received. This strategy reduces the risk of product contamination during the filling operations, helps guarantee product sterility throughout the line because of reduced vial handling, and facilitates the vial loading/unloading procedures. Given the design of the system, nested vials are spaced from one another and slightly lifted above the shelf because of the presence of the plastic material. For example, Figure 1 shows a schematic of a rack system.
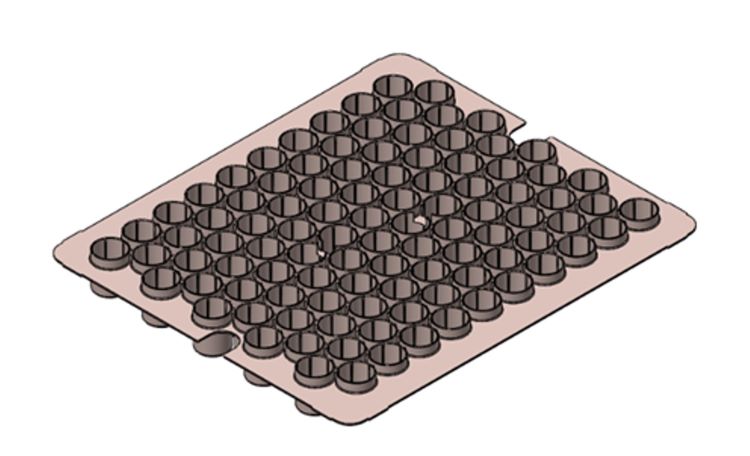
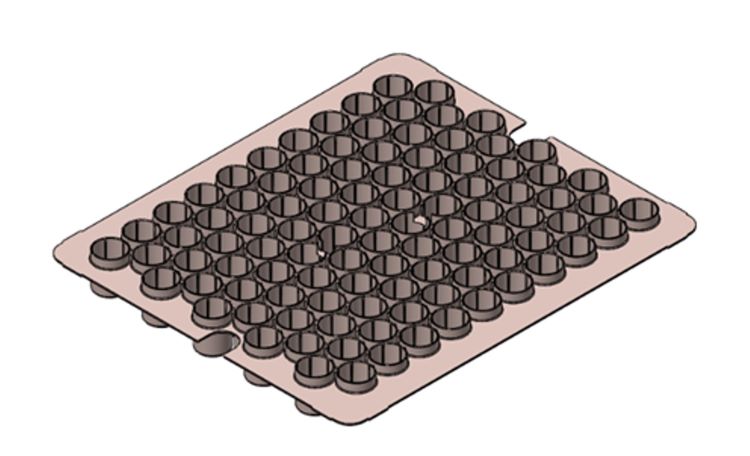
Figure 1: Schematic of the rack system. Figure reprinted with modifications (from Reference 1)
This feature impacts the heat transfer during the freezing (and the drying) phases. Compared to vials resting directly on the shelf, nested vials experience reduced heat exchange through the vial bottom because of the absence of direct contact with the shelf. The equipment-to-vial heat transfer coefficient, which considers all the heat transfer mechanisms, ie, conduction through the vial bottom and the lateral walls, results to be smaller for nested vials (48.7±5.8 W m-2K-1) compared to resting vials (77.5±7.2 W m-2K-1) during freezing. Due to the differences in the cooling protocol, the product frozen in the rack system exhibits larger ice crystals compared to resting vials.1 This feature might be desirable as it reduces the extent of the ice-water interface during freezing and, thus, the risk of interface-induced denaturation for specific biopharmaceuticals.3
How does the freezing step influence the attributes of pharmaceutical products?
During freezing, the temperature of pharmaceutical products is gradually reduced to ensure the complete solidification of the solution. This process occurs in two steps: a cooling step, where water is cooled below its freezing temperature, and a solidification step, where the phase change occurs. Solidification is initiated by a stochastic phenomenon named nucleation, ie, the formation of the first stable ice nuclei. These nuclei originate ice crystals, which grow until the entire solution is in a frozen state. The competition between nucleation and crystal growth highly impacts the morphology of ice crystals and, consequently, that of the dried products in the case of lyophilisation.4 When nucleation prevails over crystal growth, ie, ice nucleation starts at lower temperatures and/or freezing rates are high, the average ice crystals are smaller.
On the contrary, high nucleation temperatures and/or slow freezing rates lead to larger ice crystals. Due to the stochastic nature of nucleation, the product morphology can be highly variable even though all the vials undergo the same cooling protocol. As a further cause of heterogeneity, crystallisation is an exothermic phenomenon that can lead to thermal interactions between adjacent vials in highly packed arrangements. The heat released during the phase transition in a vial can alter the thermal profile of the surrounding ones, causing a delay in their nucleation.5,6
When vials are placed in a rack system, they are not in direct contact with one another, and the effect of thermal interactions can be mitigated”
When vials are placed in a rack system, they are not in direct contact with one another, and the effect of thermal interactions can be mitigated. A comparison between the nucleation time distributions measured in direct contact and nested configurations is shown in Figure 2. These distributions show a bimodal trend, indicating that some vials nucleated first and delayed the nucleation of the surrounding ones. Specifically, for the direct contact loading, the two peaks are placed around 40 and 55 minutes, respectively, while for the nested configuration at 35 and 50 minutes. In the latter case, the shift of the peaks towards shorter nucleation times and the reduced number of delayed nucleation events indicate that thermal interactions were still present. Still, their impact on neighbouring vials was mitigated. This mitigation could be beneficial in terms of batch uniformity since nucleation times are shorter and the delay effect is less relevant. In both cases, the random distribution of the nucleation times confirms the stochastic nature of nucleation.
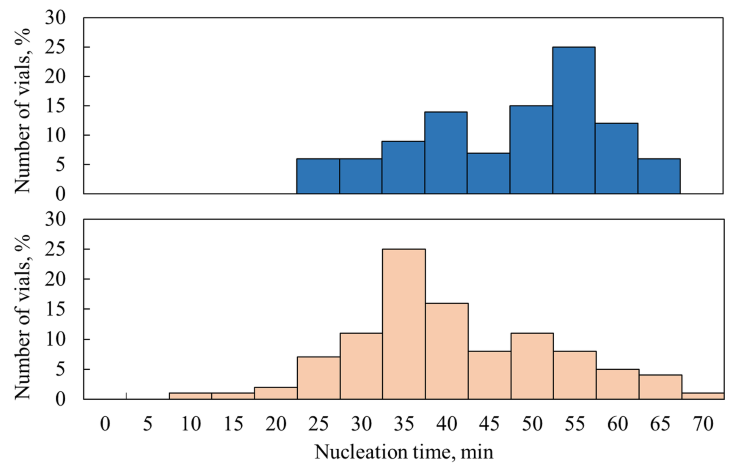
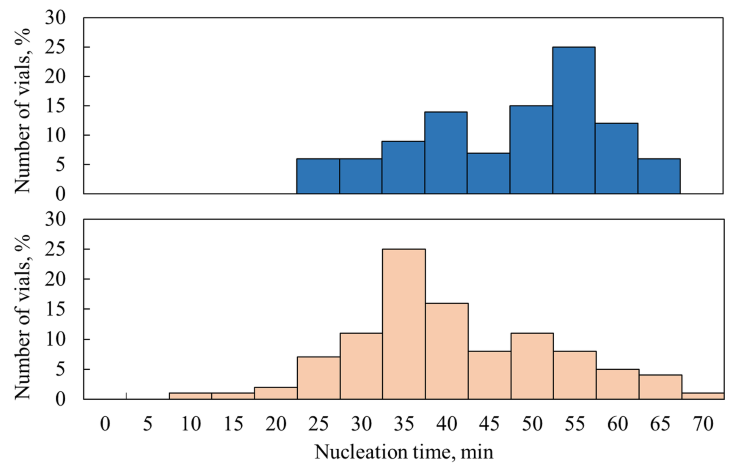
Figure 2: Nucleation time distributions, referring to the undercooling time, for the direct contact loading (blue bars) and nested loading (orange bars).
Mathematical modelling supports understanding of the freezing process
mathematical modelling could be a helpful tool for the estimation of the effects of [nucleation temperature, freezing time, and thermal interactions] on the morphology of frozen or freeze-dried products”
The effect of nucleation temperature, freezing time, and thermal interactions on the thermal history of a product is challenging to evaluate through experiments. The use of thermocouples can alter the freezing behaviour of a solution. Thus, the use of non-invasive sensors like thermal imaging cameras would be desirable. However, thermal imaging cameras cannot be applied to real case studies due to the presence of the stopper. In this context, mathematical modelling could be a helpful tool for the estimation of the effects of these phenomena on the morphology of frozen or freeze-dried products.6-8 Having a deep knowledge of the involved phenomena, mathematical modelling requires a few experimental runs to tune the parameters of the model. After that, it can be used to predict the thermal behaviour of an entire batch of vials with limited computational and experimental costs.
Figure 3 shows the distribution of nucleation temperatures computed by the model for both direct contact and nested configurations, taking also into account thermal interactions among vials. In the case of the nested loading, the nucleation temperature distribution results to be narrow and centred around -12.5°C. For the direct contact loading, the nucleation temperature distribution ranges from -5 to -17.5°C, with a peak around -10°C. These results suggest that the heat exchanged due to thermal interactions is responsible for a general increase in the nucleation temperatures and their distribution, resulting in higher heterogeneity within the batch. As expected, the presence of plastic material that separates vials in the rack system can mitigate thermal interactions, resulting in a narrower nucleation temperature distribution.
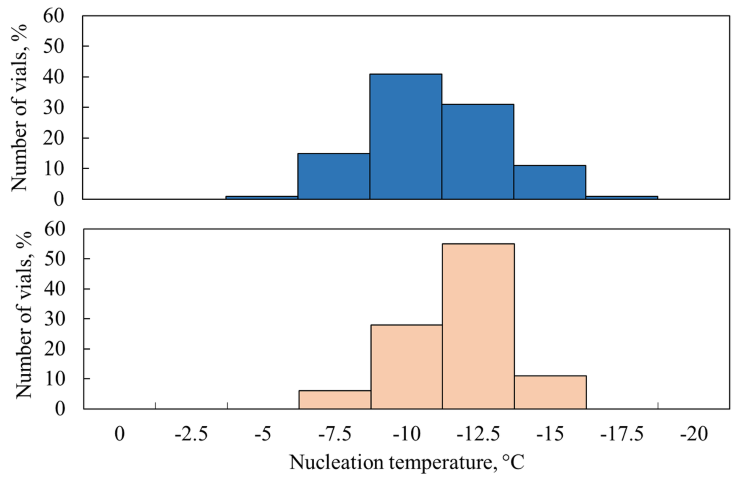
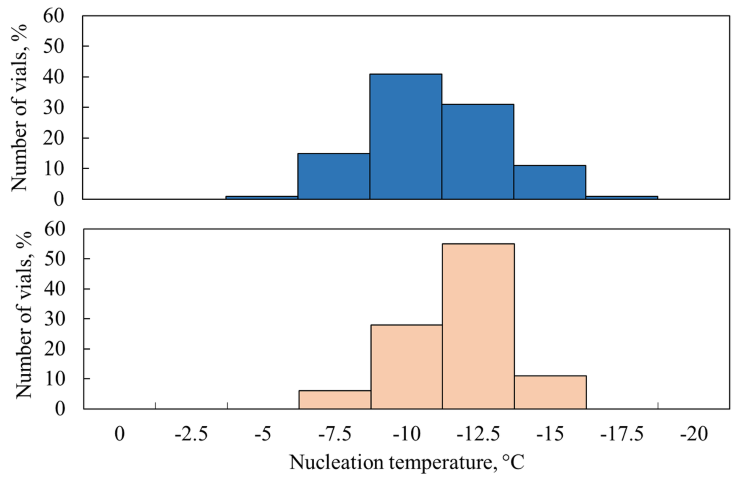
Figure 3: Nucleation temperature distributions for the direct contact loading (blue bars) and nested loading (orange bars).
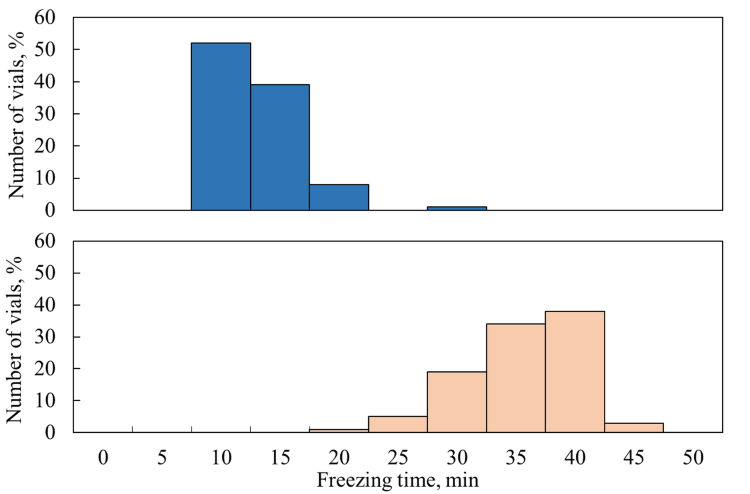
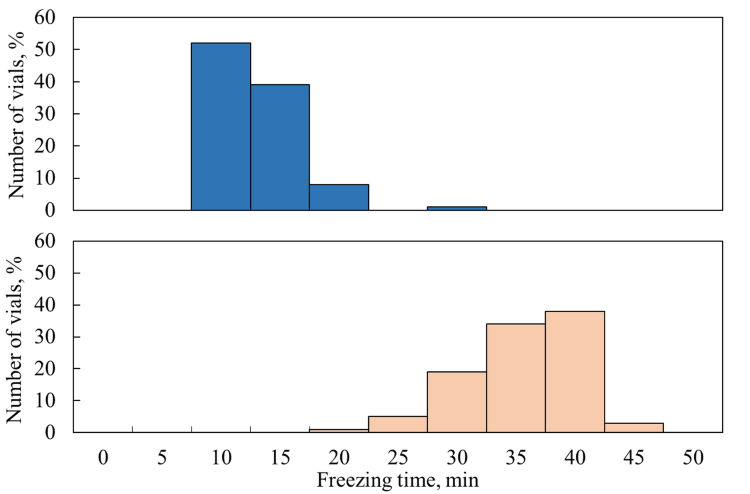
Figure 4: Freezing rate distributions for the direct contact loading (blue bars) and nested loading (orange bars).
Finally, Figure 4 reports the distributions of freezing times in the two configurations tested, showing that freezing in nested vials was about three to four times slower than in resting vials. This difference in freezing times may lead to the formation of larger ice crystals observed experimentally.1 This result can be attributed to the fact that nested vials experience slower freezing due to the absence of direct contact with the refrigerated shelf. Moreover, since thermal interactions were mitigated, the dissipation of the latent heat of solidification became less efficient. The results of the freezing model clarify the effect of different phenomena on the thermal history of pharmaceutical products frozen in vials. Specifically, they highlight several side effects of the rack system on the freezing step. The mitigation of the impact of thermal interactions ensures a more uniform nucleation temperature distribution but does not necessarily result in more uniform freezing times compared to resting vials. Nevertheless, the impact of nests on primary drying is limited, as product temperatures and drying times of nested and resting vials are comparable.2
About the authors
References
- Pisano R, Artusio F, Adami M, et al. Freeze-Drying of Pharmaceuticals in Vials Nested in a Rack System—Part I: Freezing Behaviour. Pharmaceutics. 2023;15(2):635. Available from: https://www.mdpi.com/1999-4923/15/2/635
- Artusio F, Adami M, Barresi AA, et al. The Freeze-Drying of Pharmaceuticals in Vials Nested in a Rack System—Part II: Primary Drying Behaviour. Pharmaceutics. 2023;15(11):2570.
- Arsiccio A, Giorsello P, Marenco L, Pisano R. Considerations on Protein Stability During Freezing and Its Impact on the Freeze-Drying Cycle: A Design Space Approach. J Pharm Sci. 2020;109(1):464–75.
- Searles JA, Carpenter JF, Randolph TW. The Ice Nucleation Temperature Determines The Primary Drying Rate Of Lyophilization For Samples Frozen On A Temperature‐Controlled Shelf. J Pharm Sci. 2001;90(7):860–71.
- Pisano R, Semeraro J, Artusio F, Barresi AA. Insights into Thermal Interactions in Frozen Pharmaceutical Vials: Effects on Ice Nucleation Times and Inhibition. Pharm Res. 2024;41(6):1285–97.
- Deck LT, Ochsenbein DR, Mazzotti M. Stochastic shelf-Scale Modeling Framework For The Freezing Stage In Freeze-Drying Processes. Int J Pharm. 2022;613:121276.
- Arsiccio A, Barresi AA, Pisano R. Prediction of Ice Crystal Size Distribution after Freezing of Pharmaceutical Solutions. Cryst Growth Des. 2017;17(9):4573–81.
- Capozzi LC, Pisano R. Looking inside the ‘black box’: Freezing engineering To Ensure The Quality Of Freeze-Dried Biopharmaceuticals. Eur J Pharm Biopharm. 2018;129:58–65.
Related topics
Drug Safety, Formulation, Freeze Drying, Industry Insight, Packaging, Research & Development (R&D), Therapeutics