Launching an HTS campaign to discover new antivirals
Posted: 23 May 2007 | | No comments yet
There are over 300 human viruses that have no treatment, vaccine or antiviral. Unfortunately, only sixty-two drugs are approved by the US Food and Drug Administration (FDA) for the treatment of six different viral illnesses. Of these, 45% are for the treatment of HIV/AIDS. The remaining drugs offer treatments that target hepatitis B and C, herpes, influenza, and respiratory syncytial viruses. Antiviral drugs can play a significant role in the containment of an outbreak of an emerging virus. Vaccination of individuals during an outbreak can also prove effective; however, protection of an individual from the threat may not occur for two or more weeks after the initial vaccination. Hence, only a drug can be offered as a prophylactic treatment of individuals in an endemic area.
There are over 300 human viruses that have no treatment, vaccine or antiviral. Unfortunately, only sixty-two drugs are approved by the US Food and Drug Administration (FDA) for the treatment of six different viral illnesses. Of these, 45% are for the treatment of HIV/AIDS. The remaining drugs offer treatments that target hepatitis B and C, herpes, influenza, and respiratory syncytial viruses. Antiviral drugs can play a significant role in the containment of an outbreak of an emerging virus. Vaccination of individuals during an outbreak can also prove effective; however, protection of an individual from the threat may not occur for two or more weeks after the initial vaccination. Hence, only a drug can be offered as a prophylactic treatment of individuals in an endemic area.
There are over 300 human viruses that have no treatment, vaccine or antiviral. Unfortunately, only sixty-two drugs are approved by the US Food and Drug Administration (FDA) for the treatment of six different viral illnesses. Of these, 45% are for the treatment of HIV/AIDS. The remaining drugs offer treatments that target hepatitis B and C, herpes, influenza, and respiratory syncytial viruses. Antiviral drugs can play a significant role in the containment of an outbreak of an emerging virus. Vaccination of individuals during an outbreak can also prove effective; however, protection of an individual from the threat may not occur for two or more weeks after the initial vaccination. Hence, only a drug can be offered as a prophylactic treatment of individuals in an endemic area.
The following case study presents the Southern Research Institute (SRI) approach to enabling the rapid discovery of new antiviral lead compounds for the treatment of the influenza virus1, which has also been successfully applied to SARS CoV2 and other emerging viruses. High throughput screening is a system used in pharmaceutical and biotechnology companies to screen a large number of compounds in a short period of time. In screening a library of over one million compounds, we have demonstrated that three primary areas should be taken into consideration: (1) adapting cell-based assays for HTS (2) logistics of screening and assessing the results from 1,000,000 compounds, and (3) confirming hits and obtaining early mechanism of action information.
Adapting cell-based assays for HTS
We have established a three-step protocol to automate and validate specific cell-based assays for viruses to an HTS platform:
Step 1: Determination of culture conditions to yield optimal dynamic range:
Automation of the assay to 384-well plate format requires obtaining peak cell and virus culturing conditions to achieve the optimal dynamic range. This range is measured by the difference in the fluorescent or luminescent values signal generated by a cytopathic effect (CPE) from infection with virus-infected cells versus cells alone. Generally, a HTS screen will be effective if the signal generated by the cells is five times greater than that generated by the virus-treated cells. The greater the difference between the virus and the cells, the more sensitive the assay is; and the more likely it is to capture compounds that have lower potency.
Consequently in Step 1, we evaluate various medium conditions, multiplicity of infection (MOI), and harvesting times, including the determination of the cell density and optimal virus growth conditions, and kinetics of infection. Once the optimal experimental conditions are defined, cells are seeded into 384-well plates and the following morning, are infected with virus at varying MOIs. A plate is removed after 24, 48 and 72 hours post-infection, and the end point values read. The conditions that yield the greatest dynamic range, that is, the greatest difference between infected and uninfected cells, are selected.
Step 2: Determination of compound addition parameters:
The key considerations to define the addition of compound are the concentration of the positive control drug(s), the solvent, and the compound concentration of the Master Library. The effect of each positive control drug should be assayed to determine the maximum concentration of compound that can be used with the minimal toxic effects and maximal antiviral activity. DMSO is a commonly used solvent for compound libraries; however, this solvent can negatively affect cell health or the effectiveness of the positive control compounds. To determine the maximum and optimal concentration of DMSO in the assay, varying amounts of DMSO is added to the walls in the presence of virus (quantities sufficient to result in greater than 95% cell death) with and without a control drug. Since DMSO concentrations can be problematic in obtaining the compound concentrations necessary for effective screening; its effects should be thoroughly considered prior to fixing the concentration of the Master Library.
Step 3: Determination of assay robustness:
The final step of the assay adaptation focuses on the assay robustness, which is defined as the consistency of signal for each of the experimental conditions, and the dynamic range. Individual 384-well plates are prepared which contain a single condition: cells alone, virus plus cells, cells + DMSO, or virus + control drug. An assay is considered successful when the variation for each condition is less than 10% from well to well. To confirm the consistency of the dynamic range, 384-well plates are prepared containing uninfected cells and infected cells, and the signal levels, determined. The experimental results provide data on the variability of the two conditions. These data are then subjected to a Z-value statistic to determine the number of replicates needed for each compound. For a large screen, generally greater than 50,000 compounds, the Z-value should be >0.5 indicating that each compound needs only to be tested once. These experiments are repeated at least three times to prove consistency in the assay.
Screening campaigns – logistics
Screening of a large compound library (in this case study, 1,000,000 compounds in biosafety level 2 containment) is referred to as a campaign. It has many of the logistical problems associated with a military campaign. A first and obvious consideration is to ensure an adequate supply of all materials and supplies for the entire screen. “Just in Time” is not a recommended supply strategy for a large screen. An equally important but often overlooked detail is redundancy; an inventory of replacement equipment, parts, and adequate technical staff trained for each process in the work flow. Developing a plan to account for potential equipment failures and staff emergencies will ensure smooth execution of the screen. The million compound influenza campaign was executed on the schedule given in Table 1.
Organisation of a large screening campaign into manageable tasks is something that drives the assay validation. Validation prompts questions regarding how to segment the overall process into manageable work stages as well as identifying the equipment to be used in each stage. The Southern Research High-Throughput Screening Center routinely runs 100-125 assay plates per day in 384-well format, translating to 32-40,000 compounds per batch or 25-30 batches for a 1,000,000-compound library. Many cell-based screens fail due to scale-up problems with the cells. For example, 320 million cells per day were required for the influenza assay. Sufficient incubator space for the plates should be taken into account since plates should not be stacked more than 2 high during incubation. If two batches are run each week in order to shorten the campaign, then twice as much incubator space is needed. Small groups of plates are drugged at a time to minimize the time cells spend out of the incubator. As drugging proceeds, these small groups of drugged plates are then transferred to the containment lab for virus addition. Table 2 summarises the amounts of some of the consumables that had to be organised for delivery to conduct a million compound campaign.
Effective communication and coordination among staff executing the drug addition and staff executing the virus addition steps in the process is imperative. Time from drug addition to virus addition must be as consistent as possible during the screen. Prior to the start of validation sufficient virus stocks need to be prepared and characterized. The same stock of virus should be used throughout validation and screening to minimize lot-to-lot variability.
The Southern Research Institute considers data management a priority issue – especially since every element of the process is identified and tracked by barcode. Our laboratory information management system (LIMS) generates the associations of compound plate/assay plate and tracks every step and reagent in the process. The associations from the drug delivery step are entered into Activity Base (IDBS) which is used as our data management system. Upon completion of the end point read of a batch, the technical staff notifies IT that the data is available and it is imported, analysed, reviewed by senior staff, and either verified or rejected (Figure 1). Finally, data reports are generated for verified data and rejected data is re-queued for another run.
In the influenza campaign, two batches of 100+ plates were screened per week. Cells were plated Mondays and Thursdays, drugged Tuesdays and Fridays, and read on Fridays and Mondays. A total of 27 batches (2,852 assay plates) were run over the course of 16 weeks. No batches were lost due to equipment failures or staffing problems; individual plates that were rejected during senior staff review because they failed our QC procedures were re-scheduled for screening during a subsequent batch (Figure 2).
We have since adapted this assay to a BSL-3 screen for highly pathogenic avian influenza. The strategy of developing the assay first as a BSL-2 assay and then converting to a BSL-3 strain has proven to be very effective. All the logistics and equipment issues were sorted out under normal laboratory conditions. Staff were trained and had extensive experience with the assay before moving to the much more difficult working environment of the BSL-3 lab. It is a strategy we use whenever possible in the development of BSL-3 level screens.
Hit confirmation and mechanism of action
Effective “hit” compounds are those that will prevent viral infection by 50% relative to cells infected with virus in the absence of drug. Once we identify the hits from a single dose screen, we employ two additional assays, consecutively, for additional hit assessment; (1) a dose-response with virus and toxicity and (2) plaque assay. The dose-response assay employs the identical cell-base assay and end-point reagents used in the primary screen, while the plaque assay uses a different read out such as a neutral red or an antibody based detection method. The results of these experiments reveal the potency of the hit or selective index (SI). The dose-response curves in this assay provide information on the EC50, which is the concentration of a drug which inhibits virus CPE halfway between the baseline and maximum. The dose-response curves for toxicity give an IC50. The SI50 is then calculated from the IC50/EC50. Hits with an SI50 equal to or greater than 5-10 can be further developed for mechanism of action. While low SIs may appear unattractive, studies suggest that their mechanism of action may lead to better insight into how the potency of the new scaffold can be further optimised.
Clearly, the approaches described herein are just the first steps of a challenging road that one hopes will lead to new generations of potent antivirals for the treatment of emerging viral infections. We hope the readers find this short review of our approaches helpful.
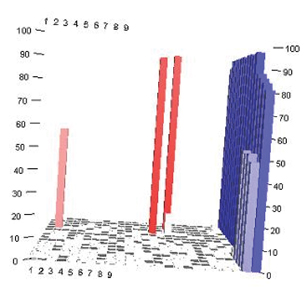
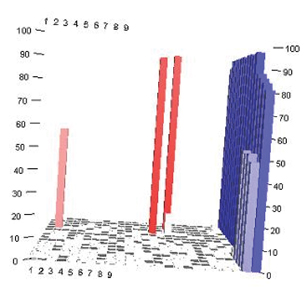
Figure 1: Depiction of One 384-Well Plate from the HTS Campaign. Controls on each plate were to the right; cell controls (blue) are in wells A24-P24, virus plus cell controls (white) are in wells A23-L23, and 40 µg/ml ribavirin (light blue) are in wells M23-P23. The remainder of the wells contained 352 testable compounds. Three potential antivirals (red) were identified on this plate. The activity of each compound was calculated in percentage (x-axis) relative to the median value for the cell controls on each plate.
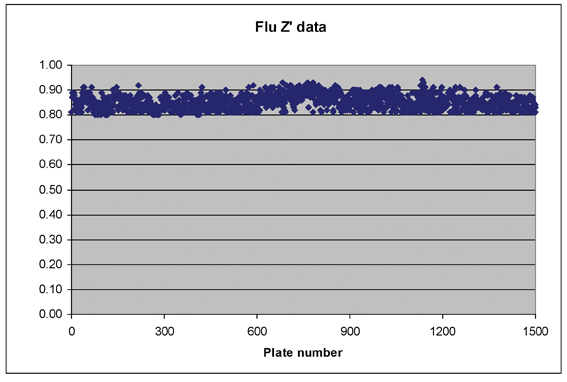
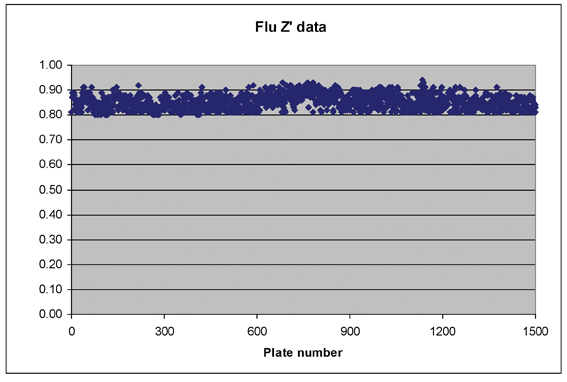
Figure 2: Quality of the million compound screen. Z-values for a representative 1,500 plates from this screen are plotted. The Z-value is a statistical measure of the quality of a plate. Control wells which included cells only and virus killed cells were included on each plate. A Z-value >_0.5 is considered good for a HTS screen; 1 is the theoretical maximum number for the calculation.
References
- Noah, J., Severson, W., Noah, D. Rasmussen, L., White, E. L. and C. B. Jonsson. (2007) A cell based luminescence assay is effective for high throughput screening of potential influenza antivirals. Antiviral Res. 73:50-59.
- Severson, W.E., Shindo, N., Sosa, M., Fletcher, T., White, L. and C. Jonsson. (2007) Development, validation and optimization of a luminescence-based high throughput screen for inhibitors of Severe Acute Respiratory Syndrome. J. Biomol. Scr. 12:33-40.
Ms. Lucile White
Ms. Lucile White is an expert in the development of methods for the purification, characterization, and assay of enzymes with over 50 publications in the area. The work at Southern Research Institute has been primarily aimed at testing the effects of drugs on target enzymes, including contract and grant work. Currently she is managing the High Throughput Screening Center at Southern Research Institute. The Institute’s CEO, Dr. Jack Secrist III, recently noted that what Ms. White has really done for the past 30 years at Southern Research Institute is to develop a career in finding ways to get things done.
Dr. Colleen Jonsson
Dr. Colleen Jonsson, Ph.D., is a Program Leader for Emerging Infectious Disease Research at the Southern Research Institute, with over 17 years of experience in the study of highly pathogenic human viruses. She has a secondary associate faculty appointment at the University of Alabama, Birmingham, in the Department of Biochemistry. Dr. Jonsson’s current research program includes investigations of hantaviruses, influenza viruses, SARS CoV, filoviruses, and retroviruses. Her studies span from basic mechanisms of viral life cycles, to the ecology and evolution of hantaviruses in Paraguay, and the discovery and mechanism of action of antivirals.