Putting complex medicines under the microscope
Posted: 10 November 2022 | Isabel Peset (Medicines Discovery Catapult), Lorna Fitzpatrick (Medicines Discovery Catapult), Martin Main (Medicines Discovery Catapult) | No comments yet
The biological characterisation of complex medicines like nucleic acid therapeutics and antibody-drug conjugates is important to understand their biodistribution and cellular delivery. Here, Isabel Peset, Lorna FitzPatrick and Martin Main from Medicines Discovery Catapult discuss the potential for using advanced light microscopy techniques.
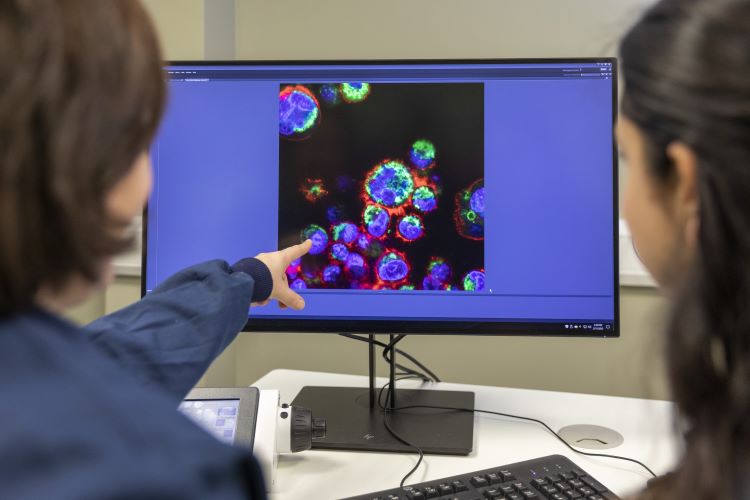
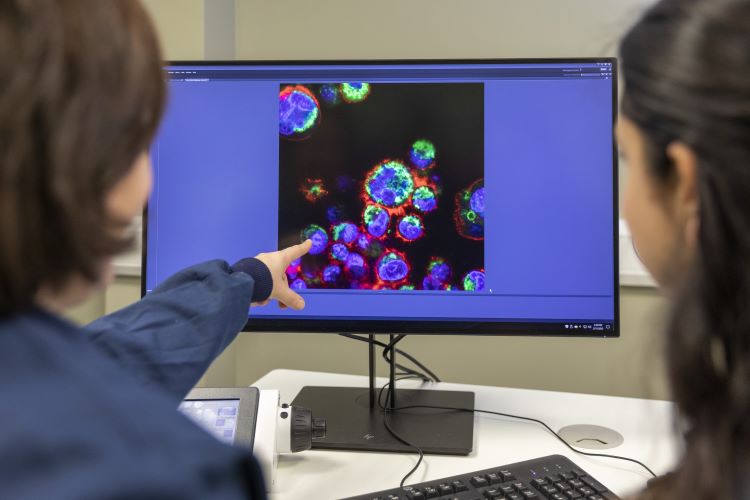
Credit: Medicines Discovery Catapult
A new wave of medicines has emerged in recent years to supplement the well-established small and large molecule platforms that have historically dominated the market. Complex medicines offer diverse therapeutic modalities, including RNA therapeutics and antibody-drug conjugates (ADCs). Advanced light microscopy techniques are one tool that can be used to understand these medicines.
The biological characterisation of complex medicines is fundamental to translate these novel therapeutics and associated drug delivery technologies into the clinic; to understand the interplay with biological systems and to evaluate new formulations. While there is a significant market opportunity in this sector, advanced analytical methods are required, and many technologies cannot provide the resolution needed.
Advanced light microscopy techniques can be used to better understand complex medicines and establish new approaches for discovery and development. Crucially, these insights can be applied across the pipeline to help more complex medicines reach the market, for patient benefit.
What constitutes a complex medicine?
With the new modalities used to treat disease and address previously undruggable targets, advanced drug delivery systems are critical to ensure targeted delivery, and to maximise safety and efficacy. It is vital to ensure adequate exposure in the target tissue, tailoring product performance to patient needs and the properties of the molecule and target.
Complex medicines will become as important as traditional small molecule drugs in the coming years, with market values of hundreds of billions of pounds1. However, the current terminology covers a broad field, with no widely agreed consensus on the definition. Medicines Discovery Catapult (MDC) released a report2 on complex medicines in 2021, proposing narrowing the definition to: ‘medicines requiring the application of novel technologies for their delivery and targeting of drugs, through the modification of an active pharmaceutical ingredient (API) or formulation, or a novel delivery route’. (Figure 1)
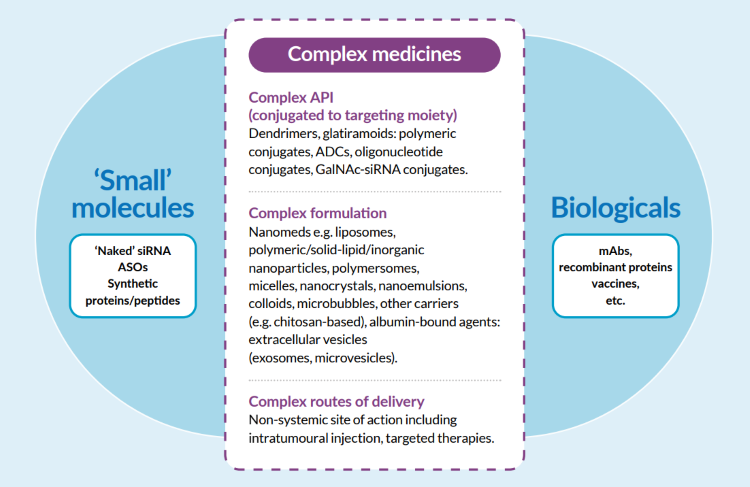
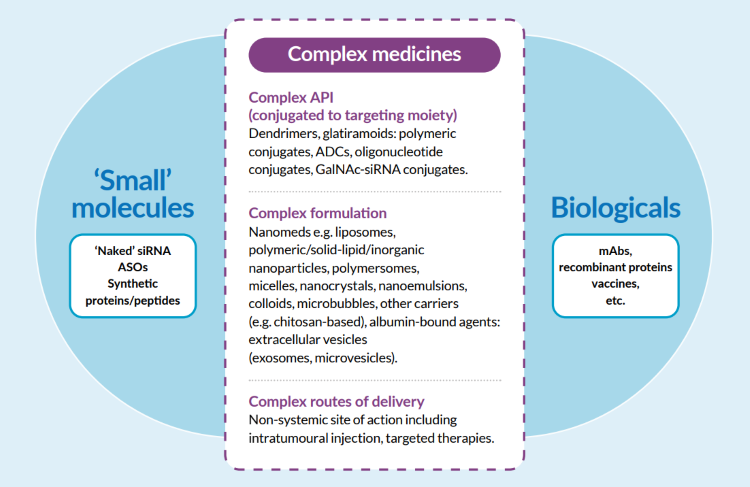
Figure 1: The proposed Medicines Discovery Catapult classification of complex medicines
Complex medicines include a variety of advanced drug chemistries and therapeutic targeting vehicles, comprising complex APIs eg, antibody-drug conjugates, oligonucleotide conjugates, complex formulations eg nanomedicines, or complex routes of delivery.
These rapidly emerging therapeutic classes offer the potential to address targets and disease indications that have been elusive to drug discovery and open a new field of industrial complex medicines discovery, development and manufacture.
Three reasons complex medicines fail at the late stages of development
Although complex medicines hold great promise to ‘drug the undruggable’ targets, additional challenges are subsequently created as there is a need to understand the properties and mechanisms of both the delivery vehicle and the cargo.
There are three primary reasons for the failure of complex medicines:
- Biodistribution – due to physiological factors, complex formulations often preferentially target the liver, and as a result, many of the clinically approved complex medicines target liver disease. Modifying or developing targeting moieties is necessary to improve extrahepatic delivery.3
- Target engagement (efficacy) – cellular uptake and cytoplasmic/nuclear delivery of cargo is a major bottleneck for clinical translation. Improved analysis pre-clinically via advanced light microscopy could help.4
- Safety – toxicity of the delivery platform and/or cargo is another common reason for the failure of complex medicines in drug discovery at both the preclinical and clinical stages.
Reviewing current approaches to drug discovery
The complexity of these medicines compared to small molecule drugs brings many challenges when developing safe and effective treatments, particularly at the early stages of drug discovery. For example, many small molecules can pass through the plasma membrane by diffusion to reach their target, whereas the tissue penetration, cellular uptake and intracellular trafficking of complex medicines can be difficult to predict. Therefore, there is a need for advanced capabilities to characterise the stability, toxicity and efficacy of both the delivery systems and cargo.
Some of the commonly used technologies for physical characterisation of a complex medicine include transmission electron microscopy (TEM), dynamic light scattering (DLS) and Zeta potential. However, these techniques provide limited information on cargo distribution on/within a delivery platform. Measuring efficacy is target-dependent and is routinely analysed via flow cytometry, western blot, qPCR, in situ hybridisation and immunohistochemistry.
Introducing the potential of advanced light microscopy
Advanced light microscopy techniques have emerged as a foundation of biomedical research, capable of visualising cellular functions at very high resolution whilst being minimally invasive to the cells or tissues of interest. Based on fluorescently labelled antibodies and drugs, the technology offers an array of benefits to drug discovery, from characterising complex cell models of disease to understanding the molecular mechanism of drug action.
In recent years, advanced light microscopy techniques have grown in popularity for characterising complex medicines. Their spatial and high-resolution capabilities provide a unique analysis tool to understand the complexity of those medicines, from their drug loading to the mechanism of action.
By combining advanced light microscopy with gene editing technologies, it is now possible to fluorescently label subcellular compartments and gain insight into complex medicine delivery and mechanism of action. (Figure 2)
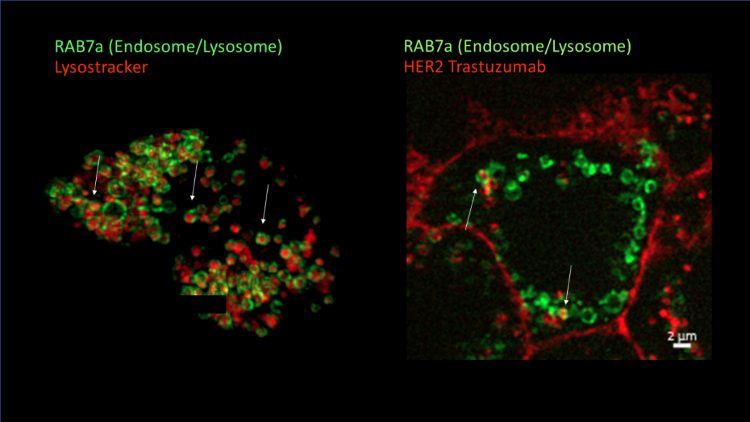
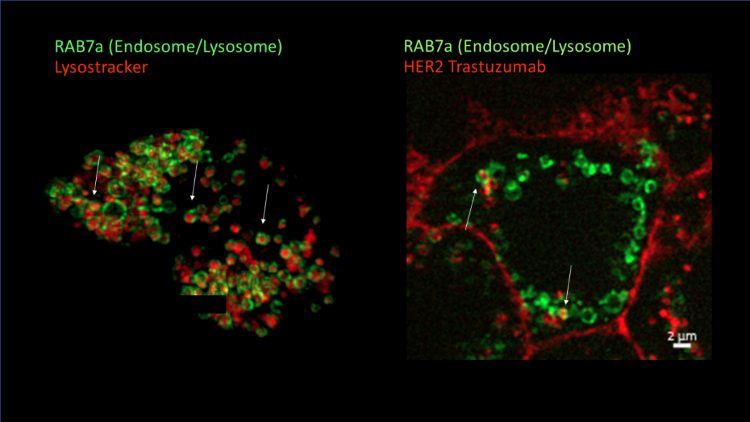
Figure 2: Super-resolution imaging of CRISPR/Cas9 reporter cell lines enables visualisation of cellular uptake of complex medicines. BT-474 cell line was gene-edited to incorporate a green fluorescent protein (GFP) tag into late endosomes/lysosomes (green). Treatment with HER2 Trastuzumab (red) results in uptake and trafficking into endosomes (white arrows indicate co-localisation)
Physical characterisation
Traditional electron microscopy gives the resolution to visualise complex medicines such as nanocarriers; however, it is not possible to see the drug cargo. Recently, single-molecule localisation microscopy technologies have provided the capability to visualise the drug delivery system and its cargo and understand their intrinsic heterogeneity and their relation to the cargo5.
Measuring efficacy
In vitro characterisation of complex medicines has traditionally been done using end-point assays to measure efficacy, which is similar to the method used for small molecules. However, those assays provide minimal information about the kinetics of cellular uptake and trafficking. One of the unique features of advanced light microscopy is the ability to use live imaging methods to visualise the cellular internalisation of complex medicines that have previously been fluorescently labelled and measure their kinetics and trafficking. This will help researchers to understand how formulation can improve cellular uptake to be more efficient.
Ex vivo tissue analysis
Toxicity and distribution in the body are vital pieces of information required for complex medicines to progress in the clinic. Ex vivo tissue analysis by advanced light microscopy (after pre-clinical imaging) can correlate tissue accumulation with penetration and specific cellular targeting using multiplex techniques. If the delivery system and/or cargo are fluorescently labelled, it will be possible also to detect the therapeutics (eg siRNA and mRNA). The spatial information that microscopy provides can unlock information about how the medicines are distributed within the tissue, intracellular detail, and which cell types are targeted.
Challenges
While the value of advanced light microscopy in understanding complex medicines has been demonstrated, it is not yet a widely used technique, as it is still low throughput and requires a specific skill set. The requirement for complex medicines to be fluorescently labelled is another limitation of the method – labelling can be a complicated process, and there is a risk that it could change the behaviour of the compounds.
However, instrument vendors are increasingly focusing on product development in advanced light microscopy and are designing devices that can provide high-resolution spatial information with higher throughput and less user intervention. Partnering with advanced light microscopy experts, like Medicines Discovery Catapult, allows drug discovery companies to introduce these techniques to their workflows, helping to drive adoption across the sector.
Future adoption of advanced light microscopy
In medicines discovery, much of the focus is placed on endpoint assays. Adopting advanced light microscopy techniques unlocks a much-needed understanding of drug mechanisms. The vast quantity of high-quality, high-resolution data that can be extracted from microscopy is helping to foster a change in methodology to steer the focus towards gathering insights at every stage of the process.
The information extracted from these powerful techniques can provide the sector with unprecedented levels of detail on the behaviour of complex medicines, giving drug innovators the confidence to make informed decisions and improve translation to the clinic. The widespread use of advanced light microscopy in medicines discovery will ultimately result in more treatments getting to patients faster.
About the authors
References
- Medicines Discovery Catapult. State of Discovery Nation 2021 – Complex Medicines: Targeted drug treatments can create a multi-billion-pound addition to the UK life sciences industry (2021) Available from: https://md.catapult.org.uk/resources/state-of-the-discovery-nation-2021/
- Medicines Discovery Catapult. Proposed definition of ‘complex medicine’: identifying technologies requiring innovation and support (2021) Available from: https://md.catapult.org.uk/wp-content/uploads/2021/04/Complex-Medicines-Report-2021.pdf
- Tewabe A, Abate A, Tamrie M, Seyfu A, Abdela Siraj E. Targeted Drug Delivery – From Magic Bullet to Nanomedicine: Principles, Challenges, and Future Perspectives. J Multidiscip Healthc. 2021 Jul 5;14:1711-1724. doi: 2147/JMDH.S313968. PMID: 34267523; PMCID: PMC8275483.
- Dominska M, Dykxhoorn D. Breaking down the barriers: siRNA delivery and endosome escape. J Cell Sci (2010) 123 (8): 1183–1189. https://doi.org/10.1242/jcs.066399
- Silva, A. M, Lázaro-Ibáñez, E., Gunnarsson, A., Dhande, A., Daaboul, G., Peacock, B., Osteikoetxea, X., Salmond, N., Friis, K. P., Shatnyeva, O., & Dekker, N. (2021). Quantification of protein cargo loading into engineered Extracellular Vesicles at single-vesicle and single-molecule resolution. Journal of Extracellular Vesicles, 10, e12130. https://doi.org/10.1002/jev2.12130
Related topics
Analytical techniques, Biopharmaceuticals, Drug Delivery Systems, Drug Development, Drug Discovery, Formulation, Research & Development (R&D)