Rapid method development to overcome challenges of bi-specific antibody purification
Posted: 27 June 2022 | Alice Harrison (Lonza), Stuart Jamieson (Lonza) | No comments yet
The landscape of biopharmaceutical manufacturing is changing, with complex molecules such as bi-specific antibodies (bsAbs) becoming increasingly prevalent. bsAbs are a large, structurally diverse family of molecules designed to recognise two targets and globally there are over 230 in development as promising therapies for cancer and other diseases. While they share structural similarities with monoclonal antibodies (mAbs), downstream purification faces challenges with bsAb-specific by-products such as mispaired chains, unwanted fragments and higher aggregation levels. Stuart Jamieson and Alice Harrison at Lonza discuss the downstream issues that development scientists encounter and the need for rapid method development.
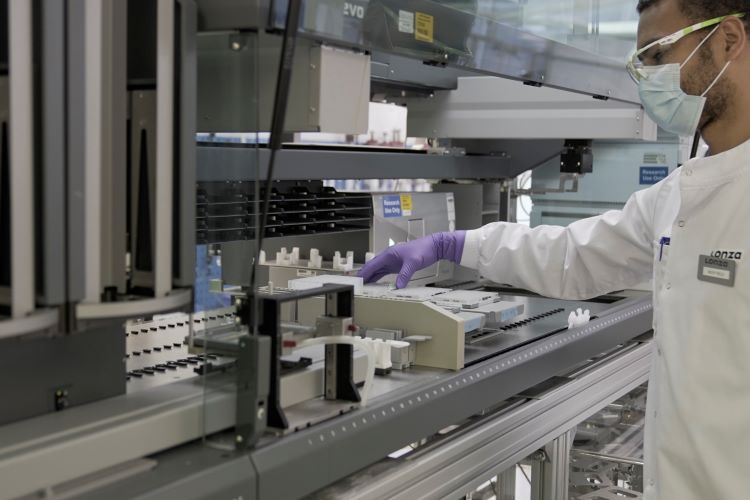
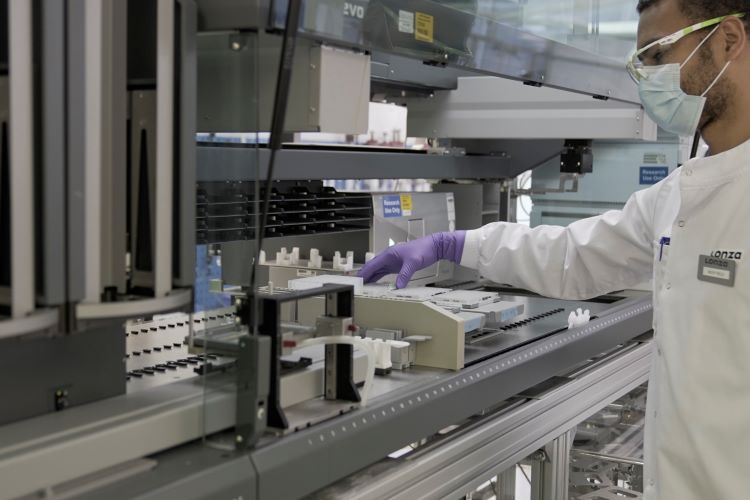
Figure 2: Automated high-throughput column chromatography screening platform
With expanding biologics pipelines encompassing an increasingly diverse range of new molecular formats, the therapeutic potential of bi-specific antibodies (bsAbs) continues to receive widespread interest.1 The majority of bsAbs consist of three or more light chain (LC) and heavy chain (HC) combinations and have the advantage of simultaneously binding two antigens via different epitopes on each antigen-binding fragment (Fab) arm (Figure 1). This dual binding enables a wide variety of applications and benefits, providing access to new therapeutic targets not available to traditional monoclonal antibodies (mAbs). Additionally, binding multiple sites facilitates more precise targeting, and results in therapies with greater potency than mAbs that are less likely to develop antibody-based clinical therapeutic resistance.2 It is therefore not surprising that more bsAbs are entering clinical development than ever before. According to the ClinicalTrials.gov trial registry, as of April 20223 there were 231 bsAbs in clinical trials and a recent industry report4 predicts the global market revenue for these products will exceed $20 billion by 2028. This has led to the development of over a 100 different bsAb formats giving rise to a wide diversity of structures within this family of molecules.5
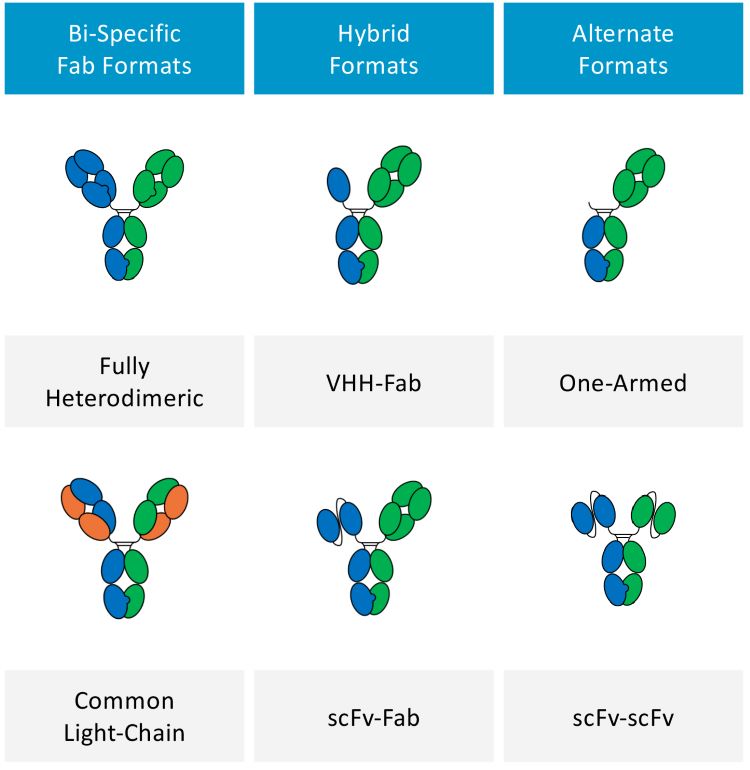
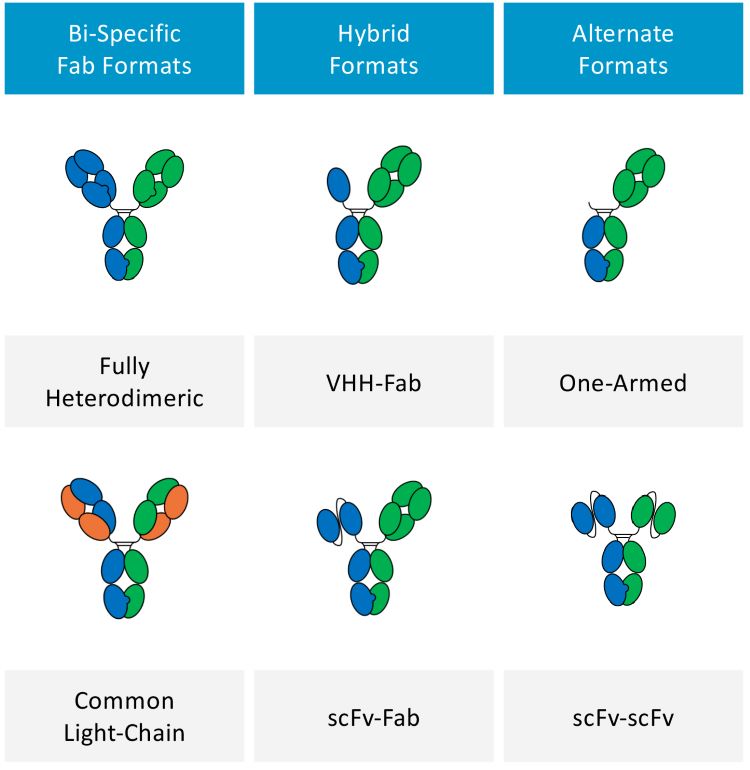
Figure 1: Example of bi-specific formats
Downstream purification approaches
Due to their structural similarities, many current bsAb downstream purification methodologies are based on established mAb purification processes, using platform affinity, charge, hydrophobicity and mixed mode-based purification. However, bsAbs present a distinct set of purification challenges due to common molecule characteristics, such as incorrect heterodimer assembly, low product yields, high aggregate concentrations and undesirable fragmentation, that are not present in mAb processes.
Integrated real-time analytics, further automation and use of data analytics to support purification development are required to increase knowledge, improve quality, ensure costeffectiveness and enhance process control”
For development purposes, bsAbs can be divided into two distinct categories depending on the presence or absence of the crystallizable fragment (Fc) region. Consistent with mAbs, bsAbs that possess an Fc region enable the use of well‑established Protein A affinity chromatography purification methods for initial product capture. Unique properties of bsAbs can, however, produce wide variability in separation performance when using Protein A resin.6 The variability can be due to molecule-specific byproducts, such as incorrect HC/LC pairs and aggregates that are usually absent or present in lower levels with mAbs; the molecule can dissociate and fragment; and even a bsAb’s isoelectric point (pI) can be different from its backbone mAb constituents. For bsAbs lacking the Fc region, alternate affinity or resin chemistry is required for the initial product capture purification stage. To overcome these additional purification challenges, problematic factors must be considered from the outset to develop bespoke scalable processes using alternative chromatography resins and strategies, such as additional affinity steps (for example, Protein G affinity or light chain capture through Protein L affinity), multiple charged and/or hydrophobicity-based purification stages, and the use of specific buffer formulations and/or pH gradients.7
High-throughput column chromatography screening
Rather than taking a platform-based approach typically used for mAbs, the customised purification that is commonly required for bsAbs can benefit from focusing on a standardised development strategy. One method is to use high-throughput (HT) chromatography screening and sample analysis to rapidly evaluate a range of purification chemistries and media designs. Purification development can then focus on selecting promising conditions for further rounds of evaluation and subsequently determine the methodology for scale-up and the final optimisation of operating parameters. Scaled‑down HT purification can be performed using automated liquid handling systems (Figure 2) available from several commercial vendors.8,9 Using these systems, chromatography screening can be performed using resin-filled filtration plates, chromatography resin‑filled tips, membrane plates or small pre‑packed micro columns (eg, OPUS® RoboColumns®, Repligen Corp). By performing HT automated scaled-down experiments, many conditions can be evaluated while minimising sample requirements and reducing time and costs.
Unlike resins in plates, micro columns are a scaled down model for larger packed chromatography columns, enabling scientists to rapidly assess a range of different resin, buffer formulation, pH and salt conditions at small scale. Rapid screening saves time and resources (Figure 3) and allows determination of the optimal combination of conditions to advance into confirmation scaleup and robustness testing. During testing, it is possible to perform step gradients and collect fractions to perform HT analytics to determine product quality attributes (PQAs) and process related impurity clearance. Such HT screening can, therefore, be used to identify capture and polishing conditions of interest, which can maximise yield, reduce aggregate and fragment levels to an acceptable limit, separate out the correct heterodimer LC/HC pairing, remove any critical process impurities, and maintain any other key PQAs.
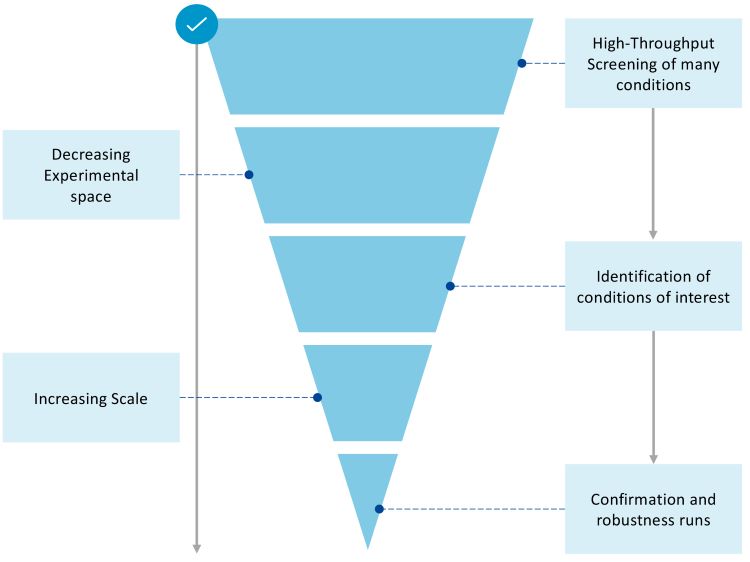
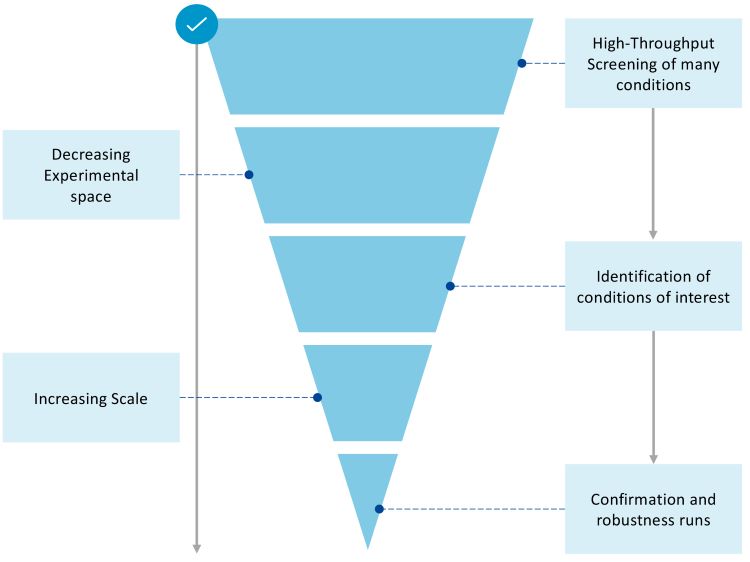
Figure 3: A schematic showing how using high-throughput screening can reduce the number of conditions of interest before scale-up
Finding the right downstream process is essential for complex molecules in early-stage development prior to scale-up. At this stage of screening, a full Design of Experiments (DoE) is not required as it is often more efficient to work to one-factor-at-a-time (OFAT) principles. The goal of early development is to identify parameters negatively impacting PQAs and quickly find promising purification methods and operating conditions that produce positive data trends and the refinement of the optimal process design. In this phase, identifying the right resins, buffer formulation and initial operating parameters is often of most interest, as these can then progress into further development prior to scaling-up for pilot and good manufacturing practice (GMP) production.
Study design of HT chromatography screening of bsAbs
In practice, an early-stage chromatography study typically involves screening a broad range of different conditions with micro columns for each capture, intermediate or polishing step. The study differs based on the components of the bsAbs; for Fc containing formats a screen of a range of Protein A resins for product capture is performed,6 whereas alternate affinity media and/or separation chemistry is used for non-Fc-containing bsAb molecules. Initial purification is followed by screening using different ion exchange (IEX) resins with a range of pH and buffer conditions, and hydrophobic interaction chromatography (HIC) resins using different salt components for intermediate or polishing steps. These steps are complimented by additional screening of mixed‑mode resins. Cation exchange (CEX) is often chosen for screening polishing steps with bsAbs as it is shown to remove bsAbs without LCs and to maintain aggregates at acceptable levels.10 HIC is also selected due to effective removal of mis-paired homodimer chains11 and good performance under mild conditions to maintain PQAs.
Analytics for assessing bsAbs from HT chromatography screening
Running multiple experiments in parallel during HT chromatography screening produces hundreds of samples for analysis, as loading, wash and elution fractions must be assessed for impurities, yield and PQAs. Analytical testing of bsAbs is also more challenging than with standard mAbs and established methods may not be suitable. There is therefore a requirement to use a toolbox of off-line analytical methods that can be optimised for suitable analytical characterisation of the bsAb of interest.
An appropriate toolbox should include traditional methods such as sodium dodecyl‑sulfate (SDS)‑gel/capillary electrophoresis for quantification and molecular weight sizing and enzyme-linked immunosorbent assays (ELISAs) for detection of residual impurities such as host cell proteins (HCPs) and ligand leachates. Commonly, reverse-phase (RP) high performance liquid chromatography mass spectrometry (HPLC-MS) and to a lesser extent size exclusion chromatography (SEC)-MS are used to determine heterodimer assembly and post-translational modifications such as glycation levels. The use of additional supporting analytics such as HT microcapillary electrophoresis and ultra-performance (UP) LC methods are also advantageous for quantification, molecular weight sizing and percentage purity analysis.
Drawing reliable conclusions from the wealth of analytical results generated, requires highly skilled data interpretation. The results generated will inform product and purification-stage limits specific for the PQAs and yield; for example, successful conditions for purification of a bsAb could be set as >90 percent correct LC/HC heterodimer levels, while maintaining aggregated at two to three percent and a bsAb yield of >85 percent.
Tables 1 and 2 show how typical analysis results from a HT chromatography screen of two CEX resins with four different pHs and four types of HIC resins with two different salts can be interpreted to determine optimum conditions to progress into scale-up for polishing a bsAb. To assess all 16 polishing conditions within this evaluation, titre and two different PQAs were chosen as the key indicators of successful purification.
Using this method to interpret the data, one promising condition was determined for CEX and two conditions of interest for HIC columns. These three conditions were further explored in a second round of HT chromatography screening, which indicated that the CEX step was sensitive to slight changes in elution conditions. The data also showed that one HIC column and salt compound combination provided the optimum balance between titre and PQAs; this data informed scaled experiments for further evaluation and method optimisation leading to the successful identification of conditions for providing specified purity levels for both PQAs in the main elution fraction and a yield of 88 percent. These results demonstrate that HT chromatography screening is useful for efficiently determining bsAbs’ polishing conditions, saving time and resources when scaling-up downstream processes.
Enhanced strategies for screening bsAbs during development
Due to the increasingly complex nature of molecules under development, it is necessary to design the analytical strategy accordingly and use methods that inform process design. Certain programmes therefore benefit from going beyond the standard analytical toolbox and supplementing with additional testing, such as HT capillary electrophoresis (CE)-MS, glycoanalytics or even high-speed ultraviolet/visible (UV/Vis) analysis to measure concentration, and dynamic light scattering (DLS) to determine molecular size and aggregate levels. The use of UPLC systems facilitate operating two flow-paths allowing the doubling of throughput or running two different methods simultaneously, for example, analysing the same set of samples by both RP and IEX, maximising productivity and data generation.
Finding the right downstream process is essential for complex molecules in early-stage development prior to scale-up”
Tools such as the Lonza High‑Throughput Impurity Detection (HTID) platform for analysis of DNA enables rapid screening of different purification conditions delivering on-time analytical data leading to timeline reduction. The rapid establishment of RP-HPLC methods for screening cell lines for correct chain assembly supports downstream development by reducing the presence of undesirable fragments. In addition, traditional physicochemical assays may not be appropriate for establishing whether impurities formed, due to the specific nature of the bsAb, impart physiologically relevant bioactivity. The potential presence of biologically active bsAb fragments relate to the structure of the molecule, the production process and its mechanism of action. As the complexity of molecules increases the probability of impurities with safety concerns, the development of biologically relevant activity assays during downstream purification and process development will become increasingly important.
As the clinical potential of novel and increasingly diverse formats of bispecific molecules continues to grow, rapid establishment of molecule-specific purification processes, by HT screening (HTS), that yield improvements in product processing and quality are becoming increasingly critical. Integrated real-time analytics, further automation and use of data analytics to support purification development are required to increase knowledge, improve quality, ensure cost-effectiveness and enhance process control.
Conclusions
When considering downstream purification methods for complex molecules such as bsAbs, it is important to remember that the process complexity required will depend on its molecular properties. While downstream methods that rapidly deliver molecules for clinical study are essential, it is also critical that these processes are fit for purpose and meet the phase-appropriate requirements for chemistry, manufacturing and controls (CMC) development, providing the basis for evolving the process where required as clinical development advances. In the absence of a true platform process for bsAbs, the use of a standardised HT purification development strategy enables exploration of a wide range of conditions. This facilitates de-risking of early-stage development timelines, the provision of bespoke downstream processes and ensures the timely delivery of a safe, effective product through its entire CMC lifecycle.
About the authors
References
- Ma J, et al. Bispecific Antibodies: From Research to Clinical Application. Front. Immunol. 2021; 12:626616. Available from: doi: 10.3389/fimmu.2021.626616.
- Thakur A, Huang M, Lum LG. Bispecific antibody-based therapeutics: Strengths and challenges. Blood Rev. 2018; 32(4):339-347. Available from: doi: 10.1016/j.blre.2018.02.004.
- Clinicaltrials.gov Database Search for Clinical Trials with bispecific antibodies | Recruiting, Not yet recruiting, Active, not recruiting, Completed, Enrolling by invitation Studies https://clinicaltrials.gov/… [Accessed 14 April 2022].
- Global Bispecific Antibody Market Opportunity, Drug Sales, Price & Clinical Trials Insight 2028 https://www.kuickresearch.com/report-global-bispecific… [Accessed 15 April 2022].
- Labrijn AF, Janmaat ML, Reichert JM, Parren PWHI. Bispecific antibodies: a mechanistic review of the pipeline. Nat Rev Drug Discov. 2019 Aug;18(8):585-608. doi: 10.1038/s41573-019-0028-1. PMID: 31175342.
- Tustian AD, Endicott C, Adams B, et al. Development of purification processes for fully human bispecific antibodies based upon modification of protein Abinding avidity. MAbs. 2016; 8(4):828-838. Available from: doi: 10.1080/19420862.2016.1160192
- Chen SW, Zhang W. Current trends and challenges in the downstream purification of bispecific antibodies. Antib Ther. 2021 May 7;4(2):73-88. doi: 10.1093/abt/tbab007. PMID: 34056544; PMCID: PMC8155696.
- Stamatis C, et al. High throughput process development workflow with advanced decision-support for antibody purification, Journal of Chromatog A. 2019; 1596:104-116. Available from doi.org/10.1016/j.chroma.2019.03.005.
- Schmidt PM, et al. A robust robotic high-throughput antibody purification platform. J Chromatog. A. 2016; 1455:9-19. Available from: doi: 10.1016/j.chroma.2016.05.076.
- 10. Wan Y, et al. Removing light chain-missing byproducts and aggregates by Capto MMC ImpRes mixed-mode chromatography during the purification of two WuXiBody-based bispecific antibodies, Protein Expression and Purification, 2020; 175 Available from doi: 10.1016/j.pep.2020.105712
- Chen T, et al. Monitoring removal of hole-hole homodimer by analytical hydrophobic interaction chromatography in purifying a bispecific antibody. Protein Expr Purif. 2019; 164:105457. Available from: doi: 10.1016/j.pep.2019.105457.