Counterfeit classifications using Raman spectroscopy and visible near-infrared (VNIR) hyperspectral imaging
Posted: 22 December 2021 | Brittany Handzo (Bristol Myers Squibb), Jeremy Peters (Bristol Myers Squibb), Ravi Kalyanaraman (Bristol Myers Squibb), Shan Xiao (Bristol Myers Squibb) | No comments yet
Colleagues Jeremy Peters, Brittany Handzo, Shan Xiao, PhD and Ravi Kalyanaraman, PhD from the Forensics and Innovative Technologies (FIT) group within Global Quality Analytical Science and Technology (GQAS&T) at Bristol-Myers Squibb, present two methods of counterfeit drug determination.
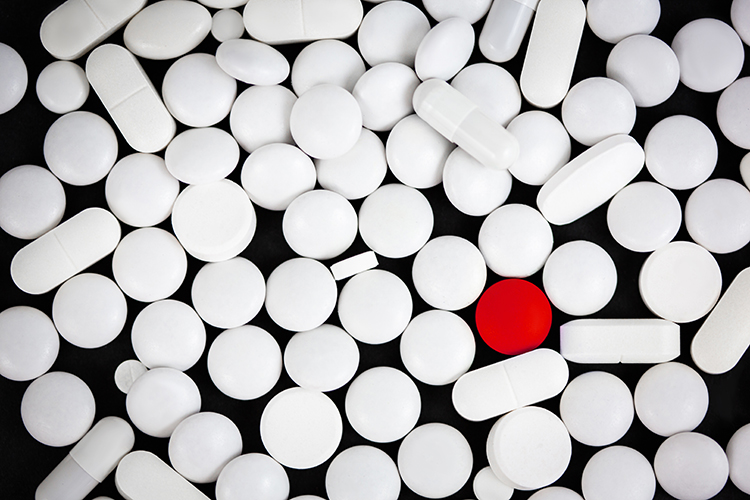
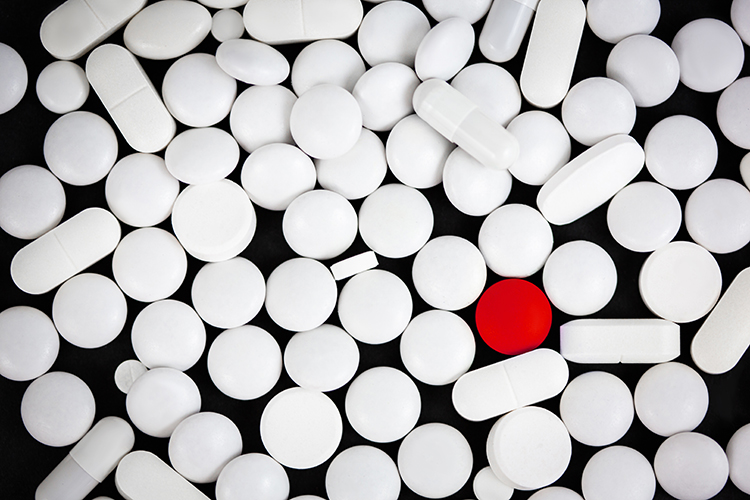
PROTECTING pharmaceutical products from counterfeiting is not only vital for any pharmaceutical company to ensure brand protection but, more importantly, to ensure the health and safety of patients.1 Several laboratories across the globe use benchtop and portable spectroscopy instruments to screen pharmaceutical counterfeits2 and one effective way to authenticate and verify the identity of pharmaceutical products is by Raman spectroscopy. Typically, a high-quality Raman spectral ‘fingerprint’ is collected for an authentic drug product. This fingerprint can then be added to a spectral library and used to screen potential suspect product. By comparing the measured Raman spectra of suspect products against the spectra of the authentic samples, counterfeit determinations can be made.3 This process is considered a simple identity testing technique. The United States Pharmacopeia (USP) compendial guideline for submitting monographs for pharmaceutical products requires more than one orthogonal method for identification testing.4,5 Therefore, in this article, we present the results from both Raman spectroscopy and hyperspectral imaging (HSI) in the visible near-infrared (VNIR, 400-1,000nm) region to correctly identify and classify four different types of counterfeits of a given branded drug product. The VNIR technique provides complementary results to Raman spectral results and confirms the classification of the counterfeits.
Raman spectroscopy is used to study fundamental modes of molecular vibrations using a monochromatic light – usually a laser. The laser light interacts with the sample, in this case a pharmaceutical drug product, and the scattered radiation is detected to gather information on the product under interrogation. The scattered light is both elastic and inelastic in nature, with the inelastic, or Raman scattering, being the one that carries information on the bond vibrations and functions used for spectral fingerprinting. This inelastic scattering is of low efficiency, which is of benefit for obtaining spectral fingerprint information without the need for sample dilution or preparation. The spectral information obtained is highly selective and the peaks are sharper, thus requiring little to no data pre-treatment. Peaks arising from functional groups such as OH, NH and CH, which are present in most pharmaceutical active ingredients and excipients, comprise the spectral fingerprint of the pharmaceutical drug product. In this study we used a benchtop FT-Raman spectrometer with a 1,064nm laser excitation with a laser power of about 1W. All spectra were acquired with 4cm-1 spectral resolution and with 256 co-added scans to obtain a good signal-to-noise (S/N) ratio.
VNIR hyperspectral imaging (HSI) is a spectroscopic technique that collects reflected energy (light) from sample surfaces at very dense and consecutive wavelengths.6 Every drug product (ex: tablet) has its own characteristic absorption and reflection of light in the VNIR spectral region of the electromagnetic spectrum. Only hyperspectral imaging can collect a detailed and specific fingerprint in this region at an extremely fast rate. The reflectance spectral fingerprint in the VNIR regions for each pixel of an image can create a map showing the quality of a given product. It can also accurately differentiate between pixels that might look the same to the human eye but can actually be quite different. One example is a counterfeit pharmaceutical where the product may look visually authentic but is chemically different. HSI data represents information that can be interpreted by powerful, yet intuitive, analytical software techniques that turn spectral libraries into analysis algorithms. Thus, HSI gives an additional dimension of information in every single pixel of a pharmaceutical product to distinguish its quality; this spectral dimension distinguishes counterfeits from authentic product. VNIR HSI instrument specifications are provided in Table 1.
Counterfeit tablet classification by Raman spectroscopy
The four known types of counterfeit tablets for a given branded drug product were initially analysed by liquid chromatography-mass spectroscopy (LC‑MS) and nuclear magnetic resonance (NMR). The chemical ingredients detected by these techniques are not provided but are referred to as Type 1 to 4 in this article. Only a few representative tablets were used for the LC-MS and NMR testing, while the remaining tablets for each counterfeit type were analysed by Fourier transform (FT)‑Raman spectroscopy. A total of 153 tablets were analysed by FT-Raman spectroscopy. Figure 1 displays one representative spectra for each counterfeit type in addition to spectra for an authentic tablet. Images of the authentic and four types of counterfeit samples are also given in Figure 1. The red spectrum represents an authentic drug product while various other coloured spectra represent the counterfeit tablets from each of the four defined groups. None of the spectra of the counterfeit tablets have identical or similar spectral fingerprints when compared against the authentic tablets. Each counterfeit type also has a unique spectral fingerprint, suggesting that they are four different types of counterfeits.
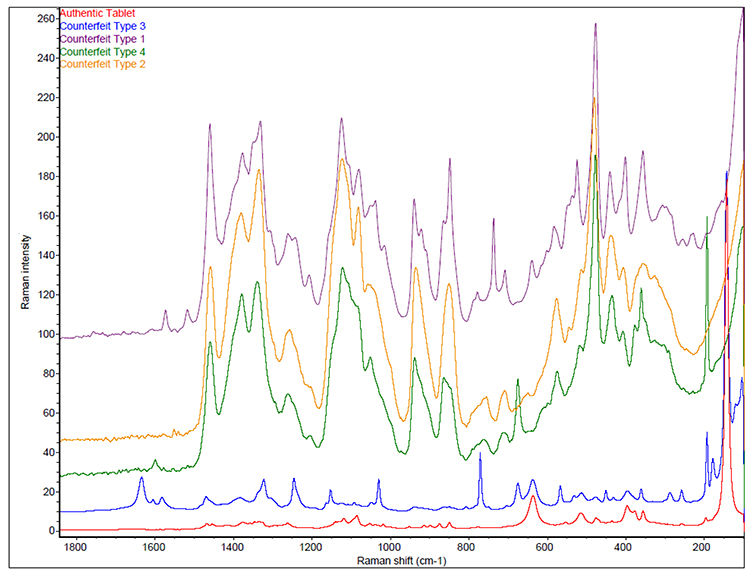
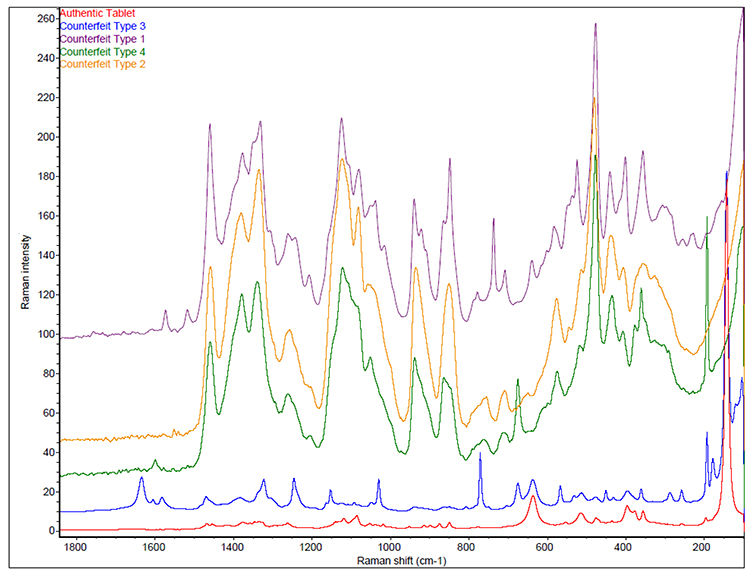
Figure 1: Representative FT-Raman spectra for authentic and each counterfeit type tablets.
A total of 143 Raman spectra were collected from all four types of counterfeits along with 10 spectra from authentic tablets. All the spectral data were pre-processed within 150 to 1,800cm-1 using standard normal variate (SNV), followed by first derivative (Savitzky-Golay) and 23-point smoothing using off-the-shelf chemometrics software. Principal component analysis (PCA) score plots of the results are shown in Figure 2, which clearly demonstrates that the four different types of counterfeits are grouped separately along with the authentic separated from the four types of counterfeits. Therefore, the Raman spectral data collected from four types of counterfeits combined with PCA analysis clearly demonstrates that this technique can be successfully used to classify counterfeits for this branded drug product.
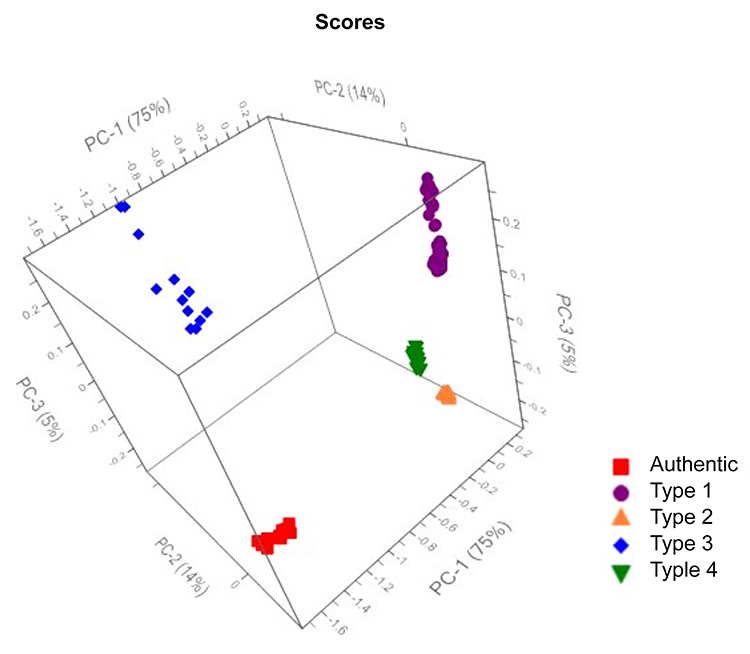
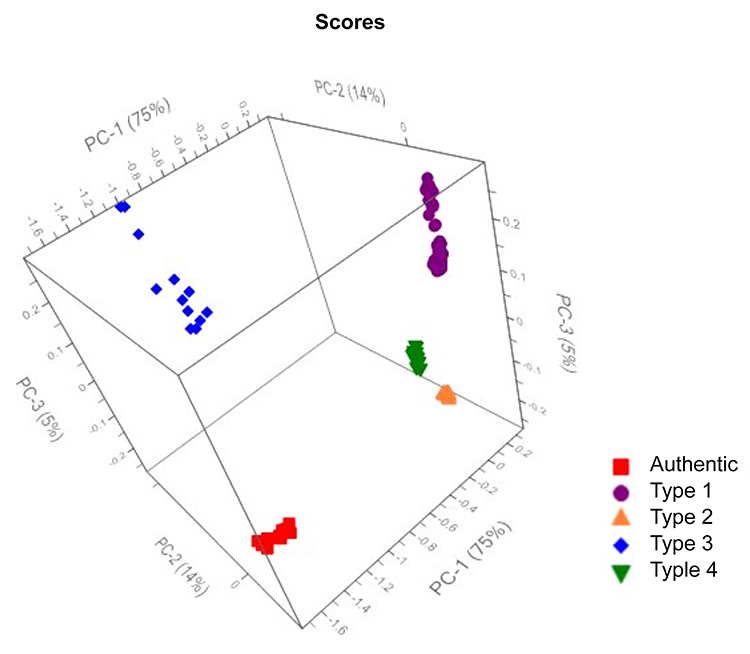
Figure 2: Principal component analysis (PCA) score plots for authentic and four different types of counterfeit tablets by Raman spectroscopy.
Counterfeit tablet classification by VNIR HSI
For VNIR HSI, a calibration dataset was created using reflectance measurements from two tablets of authentic product and two to seven tablets from each counterfeit type. The measured reflectance data were used to create a classification model for the authentic and known counterfeit types with false colour imaging for each spatial pixel. The VNIR reflected light from the tablet surface mostly captures the morphology of the tablet and not the chemical molecular fingerprint. The four types of counterfeits identified and classified by chemical fingerprinting using Raman spectroscopy possibly originates from four different types of counterfeit manufacturing operation using different manufacturing processes. Therefore, this might result in different surface properties for these four different types of counterfeit tablets. VNIR HSI results might therefore also classify them as four different types of counterfeits.
An independent sample test set consisting of 44 tablets comprised of authentic and counterfeit products were tested using the calibration model. All 44 tablets were correctly identified as the corresponding type of counterfeit, as well as the authentic, utilising the false colour imaging model. This clearly shows that the VNIR HSI technique can be used to identify and classify different types of counterfeits for a branded drug product. Figure 3 shows the calibration sample along with the test set results. The entire analysis took less than one hour, which clearly demonstrates the potential of VNIR HSI technique to rapidly screen counterfeit drugs. The false colour imaging designation for the authentic product and four different types of counterfeits are also shown in Figure 3.
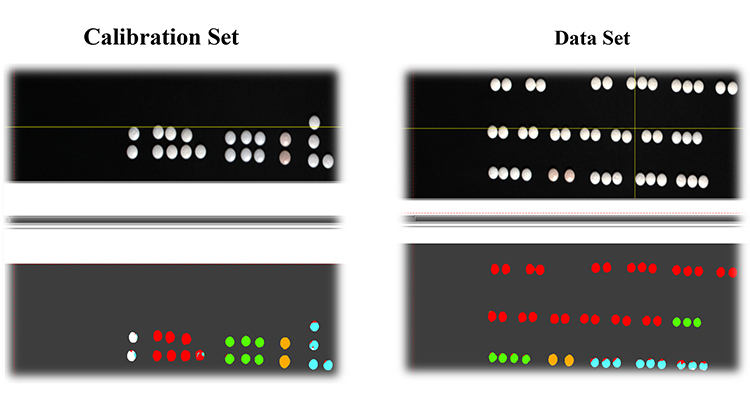
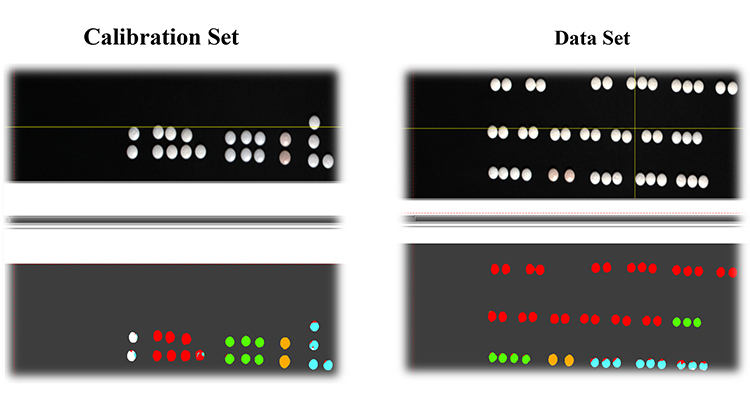
Figure 3: VNIR HSI images for authentic (white) and four different types of counterfeit tablets (Type 1 – red, Type 2 – green, Type 3 – yellow, and Type 4 – blue).
As counterfeit pharmaceuticals become an ever-increasing threat, specifically in the last decade, it has become increasingly important for pharmaceutical companies to continuously develop rapid screening tools to protect patients as well as to maintain supply chain integrity. Raman spectroscopy has shown to be a successful screening tool in detecting and combatting counterfeit pharmaceutical drugs. In this article, we have demonstrated that once initial detection of different types of counterfeits for a given branded product is confirmed using Raman spectroscopy, VNIR HSI can possibly replace this and be implemented successfully for rapid detection of pharmaceutical counterfeits.
About the authors
References
- European Medicines Agency website, Available from: https://www.ema.europa.eu/en/human-regulatory/overview/public-health-threats/falsified-medicines-overview
- Handzo B, Luczak A, Huffman S, et al. Benchtop and Portable Raman Spectrometers to Screen Counterfeit Drugs, American Pharmaceutical Review, 2020 April, vol. 23, issue 3, 38 – 43.
- Kalyanaraman R, Ribick M, Dobler G. Portable Raman Spectroscopy for Pharmaceutical Counterfeit Detection, European Pharmaceutical Review, 2012, vol. 17, issue 5, 35 – 39.
- United States Pharmacopeia, , General Notices and Requirements, Section 5.40, Identification Test.
- United State Pharmacopeia Guideline for Submitting Requests for Revision to USP-NF, Section 4, Identification.
- Jia J, Wang Y, Chen J, et al. Status and application of advanced airborne hyperspectral imaging technology: A review, Infrared Physics and Technology, January 2020, vol. 104, 1 – 16.