Drug discovery assays for the histone deacetylase class of enzymes
Posted: 18 December 2012 |
The histone deacetylase (HDAC) class of enzyme are a group of conserved enzymes known for their ability to remove acetyl groups from lysine residues on histone tails. Since aberrant HDAC enzyme expression is observed in various diseases, there is increasing interest in finding small molecules which function as HDAC enzyme inhibitors. This article reviews the various biochemical assays available for monitoring HDAC enzyme activity that have been validated for use in High Throughput Screening. The assays referred to are compatible with standard microtitre plates (96 and 384 well format) and make use of absorbance, luminescence and fluorescence detection methods.
The histone deacetylase class of enzymes: The histone deacetylase (HDAC) class of enzymes are involved in many biological pathways and one of their best known properties is their ability to remove acetyl groups from lysine residues on amino-terminal histone tails. Thus far, 18 HDAC enzymes have been identified which are divided into zinc dependent and NAD dependent enzymes. The Class I HDAC enzymes include the zinc dependent HDACs 1, 2, 3, and 8 and consist of 350-500 amino acid residues. The Class II HDAC enzymes are also zinc dependent but are larger, consist of about 1,000 amino acid residues and are subdivided into Class IIa (HDAC4, 5, 7, and 9) and Class IIb (HDAC6 and HDAC10) enzymes. The Class I and Class II HDAC enzymes can be inhibited by trichostatin A (TSA) and this inhibitor is often used as a reference to bench-mark their assays. The Class III HDAC enzymes are the sirtuin enzymes (SIRT1-7) and are NAD-dependent. This class of enzymes is not sensitive to TSA but can be inhibited for example by nicotinamide.
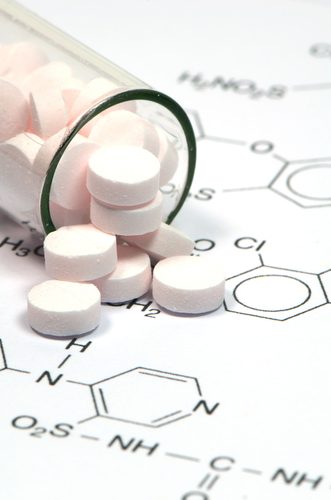
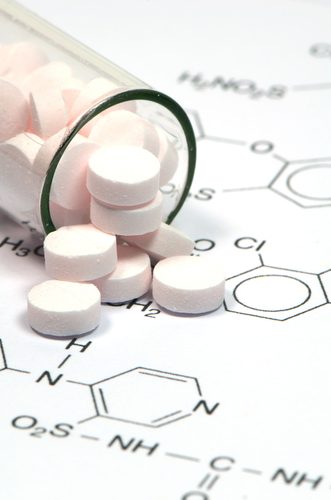
The histone deacetylase (HDAC) class of enzyme are a group of conserved enzymes known for their ability to remove acetyl groups from lysine residues on histone tails. Since aberrant HDAC enzyme expression is observed in various diseases, there is increasing interest in finding small molecules which function as HDAC enzyme inhibitors. This article reviews the various biochemical assays available for monitoring HDAC enzyme activity that have been validated for use in High Throughput Screening. The assays referred to are compatible with standard microtitre plates (96 and 384 well format) and make use of absorbance, luminescence and fluorescence detection methods.
The histone deacetylase class of enzymes
The histone deacetylase (HDAC) class of enzymes are involved in many biological pathways1 and one of their best known properties is their ability to remove acetyl groups from lysine residues on amino-terminal histone tails2-4. Thus far, 18 HDAC enzymes have been identified which are divided into zinc dependent and NAD dependent enzymes5. The Class I HDAC enzymes include the zinc dependent HDACs 1, 2, 3, and 8 and consist of 350-500 amino acid residues6. The Class II HDAC enzymes are also zinc dependent but are larger, consist of about 1,000 amino acid residues7 and are subdivided into Class IIa (HDAC4, 5, 7, and 9) and Class IIb (HDAC6 and HDAC10) enzymes. The Class I and Class II HDAC enzymes can be inhibited by trichostatin A (TSA)8,9 and this inhibitor is often used as a reference to bench-mark their assays. The Class III HDAC enzymes are the sirtuin enzymes (SIRT1-7) and are NAD-dependent1,10. This class of enzymes is not sensitive to TSA but can be inhibited for example by nicotinamide.
Histone deacetylase inhibitors
As the HDAC enzymes are implicated in various diseases and cancer11, there is significant interest in discovering HDAC inhibitors (HDACi’s) that could be used as potential therapeutic agents. In addition, the use of HDACi’s as part of combination therapy in the treatment of various cancer diseases has shown to be promising1,12. HDACi’s are classified according to their chemical structures: hydroxamic acids, cyclic peptides, short chain fatty acids, benzamides and electrophilic ketones13,14. One of the first identified HDAC’s was trichostatin A (TSA), an antifungal antibiotic2,10,15 which was isolated from Streptomyces platensis culture broth16. It is a reversible HDAC which has an effect at nanomolar concentrations17,18 and has been reported to prevent tumorigenesis and metastasis2,19. TSA is a representative of the hydroxamic acids and is often used to investigate histone acetylation2 as its mechanism of inhibition is known to be due to chelating the HDAC enzyme zinc ion4,20. Due to this mechanism, TSA is a Class I and II HDACi but not an inhibitor for Class III HDACs since these enzymes do not contain a zinc ion.
Two HDACi’s have been approved by the FDA for clinical use. The first was SAHA (Vorinostat), a hydroxamic acid, for treatment of cutaneous T-cell lymphoma2 and the second is the natural product Romidepsin (depsipeptide) that was isolated from Chromobacterium violaceum also used to treat cutaneous T-cell lymphoma15. Since most HDACi’s are not selective towards the specific HDAC enzymes (i.e. are ‘pan-active’ inhibitors), identifying selective HDACi’s is a major challenge11,15,20 and suitable screening compatible assays are required for these purposes and these are described below.
High Throughput Screening compatible HDAC assays for drug discovery
The initial approach for monitoring HDAC enzyme activity made use of radio-labelled histones as the substrate21 or unlabelled, acetylated histone peptides that required resolution by HPLC22. These assays are relatively low throughput and for modern day drug discovery projects, alternative assays have been developed that are microtitre plate based. Many of these assays make use of peptide substrates containing an ε-acetylated lysine residue that contain the following specific features that allow the detection of HDAC enzyme activity, and these include (i) a peptide substrate coupled to a suitable leaving group that would allow the quantification of substrate that has been turned over by the HDAC enzyme, (ii) a peptide substrate being biotinylated and an antibody that recognises the acetylated / deacetylated lysine residue to quantify how much of the substrate has been turned over by the HDAC enzyme or (ii) a fluorolabelled ligand that binds tagged HDAC enzymes whose displacement can be used to quantify their affinity for the HDAC enzyme activity. Below are more details pertaining to these HDAC assays.
- Functional colorimetric HDAC assay
The colorimetric HDAC assay makes use of chromogenic peptide substrates that contains an ε-acetylated lysine residue. When an HDAC enzyme acts upon the substrate and the sidechain of an ε-acetylated lysine residue is deacetylated, it becomes susceptible to further degradation by an enzyme in the developer reagent. The action of the protease enzyme within the developer reagent results in the release of the 4-nitroanilide chromophore that is detected by measuring the absorbance of the reaction at 405 nanometres. As this is a colorimetric based assay, it is generally of low sensitivity and prone to optical interference23. In addition, as this assay is enzyme coupled, a suitable counter assay is required to ensure any apparent inhibitors that are identified are genuine HDAC enzyme inhibitors and not of the enzyme in the developer reagent. - Functional fluorescence intensity HDAC assays
This assay is similar in principle to the colorimetric assay described above but it involves the replacement of the chromogenic group with one that is fluorogenic. The detection of the product can be performed by measuring the increase in fluorescence after appropriate excitation. These fluorogenic assays are more sensitive and less prone to optical interference from compounds than their counterpart colorimetric based assays and therefore offer significant advantages24,25. These assays are also enzyme coupled, therefore a suitable counter assay is required to ensure any apparent inhibitors that are identified are genuine HDAC enzyme inhibitors and not of the enzyme in the developer reagent. - Functional luminescence HDAC assays
This assay is also similar to those described above (colorimetric and fluorescence intensity) but make use of an aminoluciferin labelled ε-acetylated lysine peptide substrate. When the substrate is acted upon by HDAC enzymes so as to undergo deacetylation, the product becomes susceptible to the protease cleavage within the developer reagent which results in the release of aminoluciferin. This aminoluciferin is the substrate for a luciferase enzyme (also in the developer reagent) resulting in a detectable luminescence signal26. This assay is also enzyme coupled, therefore a suitable counter assay is required to ensure any apparent inhibitors that are identified are genuine HDAC enzyme inhibitors and not of the enzymes in the developer reagent. - Functional luminescent/fluorescent HDAC assays
The assay is different from those described above as it does not make use of a coupled assay. The assay makes use of a biotinylated ε-acetylated lysine peptide substrate that is acted upon by the HDAC enzymes. Upon deacetylation of the substrate, an anti-ε- deacetylated-lysine-antibody (conjugated to an acceptor bead) can bind the product whilst a streptavidin conjugated donor bead binds the biotinylated peptide (regardless of whether it is acetylated / deacetylated). As this is a proximity based assay, the donor and acceptor beads will only be in suitably close proximity when the biotinylated peptide is deacetylated. The detection of product is made by excitation of the donor bead at 680 nano – metres from which singlet oxygen is released that diffuses to the acceptor bead, excites the fluorophore within it resulting in the emission of light at 615 nanometres27. - Binding fluorescence polarisation HDAC assay
This is a competitive binding assay that makes use of a fluoro-labelled ligand that binds the HDAC enzyme. Upon its displace ment from the HDAC enzyme, its fluorescence polarisation changes significantly. This assay is capable of detect – ing compounds that displace the fluorolabelled ligand from the HDAC enzyme and thus provide information regarding their affinity for the HDAC enzyme28. - Binding time-resolved fluorescence resonance HDAC assays
These are also competitive binding assays that make use of fluoro-labelled ligands (acceptors) that bind HDAC enzymes. However, as these are proximity based assays, they require tagged HDAC enzymes (e.g. glutathione S-transferase) that can bind a Europium-labelled anti-enzyme tag antibody (donor). If the donor and acceptor are in close proximity and excited at 340 nanometres, the Europium undergoes excitation and emits at 615 nanometres. If the acceptor is in close proximity, the fluorophore undergoes excitation at 615 nanometres and emits at 665 nano – metres. This assay is capable of detecting compounds that displace the fluorolabelled ligands from the HDAC enzyme and thus provide information regarding their affinity for the HDAC enzyme29,30.
Conclusion
With the technological advances in HTS, the screening large compounds libraries are usually not a bottle-neck in the drug discovery process. In contrast, assay development can still be a relatively lengthy process, especially for novel targets. In the case of the kinase enzymes, more than 20 different assay formats are available for use in drug discovery with each assay having its own advantages and disadvantages31. Although the HDAC enzymes have been implicated in a number of disease states, they are still underexplored with respect to the discovery of inhibitors for clinical use. Many of the HDAC assays described in this article are available as kits from commercial suppliers and they have been validated for HTS and drug discovery purposes. It is anticipated that the HDAC assays described in this article can collectively be used to allow the identification of chemical starting points from HTS campaigns. Although there may be a preference to use one of the assays described in this article for drug discovery projects, the range of assays described in this article can be employed as counter assays such that the activities of any inhibitors can be confirmed in other assay formats and provide confidence that are not due to artefacts.
References
- Bertrand, P. Inside HDAC with HDAC inhibitors. European Journal of Medicinal Chemistry, 45 (2010) 2095-2116
- Gallinari, P., Di Marco, S., Jones, P., Pallaoro, M., Steinkühler, C. HDACs, histone deacetylation and gene transcription: from molecular biology to cancer therapeutics. Cell Research, 17 (2007) 195-211
- Abel, T., Zukin, R.S. Epigenetic targets of HDAC inhibition in neurodegenerative and psychiatric disorders. Current Opinion in Pharmacology, 8 (2008) 57-64
- Smith, K.T., Workman, J.L. Histone deacetylase inhibitors: Anticancer compounds. The International Journal of Biochemistry & Cell Biology, 41 (2009) 21-25
- Glaser, K.B. HDAC inhibitors: Clinical update and mechanism-based potential. Biochemical Pharma – cology, 74 (2007) 659-671
- Spange, S., Wagner, T., Heinzel, T., Krämer, O.H. Acetylation of non-histone proteins modulates cellular signalling at multiple levels. The International Journal of Biochemistry & Cell Biology, 41 (2009) 185-198
- Guardiola, A.R., Yao, T. Molecular cloning and characterization of a novel histone deacetylase HDAC10. The Journal of Biological Chemistry, 277/5 (2002) 3350-3356.
- Marks, P.A., Miller, T., Richon, V.M. Histone deacetylases. Current Opinion in Pharmacology, 3 (2003) 344-351
- Ropero, S., Esteller, M. The role of histone deacetylases (HDACs) in human cancer. Molecular Oncology, 1 (2007) 19-25
- Thiagalingam, S., Cheng, K., Lee, H.J., Mineva, N., Thiagalingam, A., Ponte, J.F. Histone deacetylases: Unique players in shaping the epigenetic histone code. Annals of the New York Academy of Sciences, 983 (2003) 84-100
- Segré, C.V., Chiocca, S. Regulating the regulators: The post-translational code of class I HDAC1 and HDAC2. Journal of Biomedicine and Biotechnology, 2011 (2010)
- Pollock, R.M., Richon, V.M. Epigenetic approaches to cancer therapy. Drug Discovery Today: Therapeutic Strategies, 6/2 (2009)
- Graham, J.S., Kaye, S.B., Brown, R. The promises and pitfalls of epigenetic therapies in solid tumors. European Journal of Cancer, 45 (2009) 1129-1136
- Jones, P., Altamura, S., Chakravarty, P.K., Cecchetti, O., de Francesco, R., Gallinari, P., Ingeito, R., Meinke, P.T., Petrocchi, A., Rowley, M., Scarpelly, R., Serafini, S., Steinkühler, C. A series of novel, potent, and selective histone deacetylase inhibitors. Bioorganic & Medicinal Chemistry Letters, 16 (2006) 5948-5952
- McLaughlin, F., La Thangue, N.B. Histone deacetylase inhibitors open new doors in cancer therapy. Biochemical Pharmacology, 68 (2004) 1139-1144
- Somech, R., Izraeli, S., Simon, A.J. Histone deacetylase inhibitors – a new tool to treat cancer. Cancer Treatment Reviews, 30 (2004) 461-472
- Luo, R.X., Dean, D.C. Chromatin remodeling and transcriptional regulation. Journal of the National Cancer Institute, 91/15 (1999)
- Chang, J., Varghese, D.S., Gilliam, M.C., Pexton, M., Modi, B., Schlitz, R.L., Girard, L., Martinez, E.D. Differential response of cancer cells to HDAC inhibitors trichostatin A and depsipeptide. British Journal of Cancer, 106 (2012) 116-125
- Mogal, A., Abdulkadir, A. Effects of histone deacetylase inhibitor (HDACi); trichostatin-A (TSA) on the expression of housekeeping genes. Molecular and Cellular Probes, 20 (2006) 81-86
- Witt, O., Deubzer, H.E., Milde, T., Oehme, I. HDAC family: What are the cancer relevant targets? Cancer Letters, 277 (2009) 8-21
- Kölle, D., Brosch, G., Lechner, T., Lusser, A., Loidl, P. Biochemical Methods for Analysis of Histone Deacetylases. Methods, 15 (1998) 323–331
- Pacholec, M., Bleasdale, J.E., Chrunyk, B., Cunningham, D., Flynn, D., Garofalo, R.S., Griffith, D., Griffor, M., Loulakis, P., Pabst, B., Qiu, X., Stockman, B., Thanabal, V., Varghese, A., Ward, J., Withka, J., Ahn, K. SRT1720, SRT2183, SRT1460, and Resveratrol Are Not Direct Activators of SIRT1. Journal of Biological Chemistry, 285 (2010) 8340-8351
- Liu, Y., Liggitt, D., Fong, S., Debs, R.J. Systemic coadministration of depsipeptide selectively targets transfection enhancement to specific tissues and cell types. Gene Therapy, 13 (2006) 1724-1730
- Wegener, D., Hildmann, C., Riester, D., Schober, A., Meyer-Almes, F.J., Deubzer, H.E., Oehme, I., Witt, O., Lang, S., Jaensch, M., Makarov, V., Lange, C., Busse, B., Schwienhorst, A. Identification of novel smallmolecule histone deacetylase inhibitors by mediumthroughput screening using a fluorigenic assay. Biochemical Journal, 413 (2008) 143-150
- Gul, S., Gribbon, P. Exemplification of the challenges associated with utilising fluorescence intensity based assays in discovery. Expert Opinion On Drug Discovery, 5 (2010) 681-690.
- Halley, F., Reinshagen, J., Wolf, M., Niles, A.L., Evans, N.J., Wagner, J.M., Jung, M., Gribbon, P., Gul, S. A bioluminogenic HDAC activity assay – validation and screening. Journal of Biomolecular Screening, 16 (2011) 1227-1235
- Wigle, T.J., Herold, J.M., Senisterra, G.A., Vedadi, M., Kireev, D.B., Arrowsmith, C.H., Frye, S.V., Janzen, W.P. Screening for inhibitors of low-affinity epigenetic peptide-protein interactions: an AlphaScreen-based assay for antagonists of methyl-lysine binding proteins. Journal of Biomolecular Screening, 15 (2010) 62-71
- Mazitschek, R., Patel, V., Wirth, D.F., Clardy, J. Development of a fluorescence polarization based assay for histone deacetylase ligand discovery. Bioorganic & Medicinal Chemistry Letters, 18 (2008) 2809-2012
- Marks, B.D., Fakhoury, S.A., Frazee, W.J., Eliason, H.C., Riddle, S.M. A substrate-independent TR-FRET histone deacetylase inhibitor assay. Journal of Biomolecular Screening, 10 (2011) 1247-1253
- Robers, M.B., Loh, C., Carlson, C.B., Yang, H., Frey, E.A., Hermanson, S.B., Bi, K. Measurement of the cellular deacetylase activity of SIRT1 on p53 via LanthaScreen® technology. Molecular Biosystems, 7 (2011) 59-66
- Ma, H., Deacon, S., Horiuchi, K., The challenge of selecting protein kinase assays for lead. Expert Opinion On Drug Discovery, 3 (2008) 607-621
About the authors
Sheraz Gul is Vice President and Head of Biology at European ScreeningPort, Hamburg, Germany. He is responsible for the manage – ment and development of Medium and High Throughput Screening activities for academic partners across Europe. He has 12 years research and development experience in both academia (University of London) and industry (GlaxoSmithKline Pharmaceuticals). This has ranged from the detailed study of catalysis by biological catalysts (enzymes and catalytic antibodies) to the design and development of assays for High Throughput Screening for the major biological target classes. He is the co-author of numerous papers, chapters and the Enzyme Assays: Essential Data handbook.
Gesa Witt finished her undergraduate studies in Biotechnology at the University of Applied Sciences, Hamburg in 2010. Her studies were completed by a six month internship in Switzerland. She continued her studies by starting a Master’s Program in Pharmaceutical Biotechnology at the University of Applied Sciences in Hamburg which was completed in 2012. As part of the Master’s Program, she worked on HDAC drug discovery at the European ScreeningPort during 2012.