‘Magic bullets’ for all: democratising biologics by rethinking antibody development, manufacturing and delivery
Posted: 31 August 2021 | Brian Finrow (Lumen Bioscience), Craig Behnke (Lumen Bioscience) | No comments yet
Antibody therapeutics revolutionised how diseases like cancer are treated in the developed world, but their high manufacturing cost and cumbersome distribution and administration leave them out of reach for most. In this guest article, Lumen Bioscience’s CEO, Brian Finrow, and EVP of Production & Development, Craig Behnke, discuss how new technologies are making mass-market antibody drugs feasible for ultra-prevalent diseases like COVID-19 for the first time.
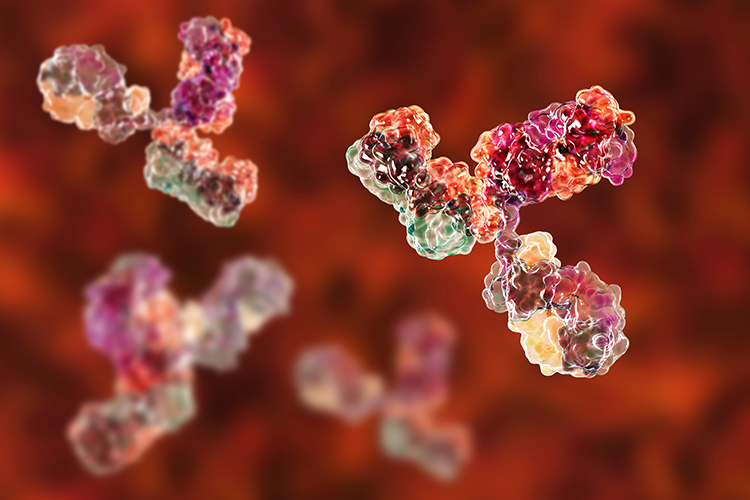
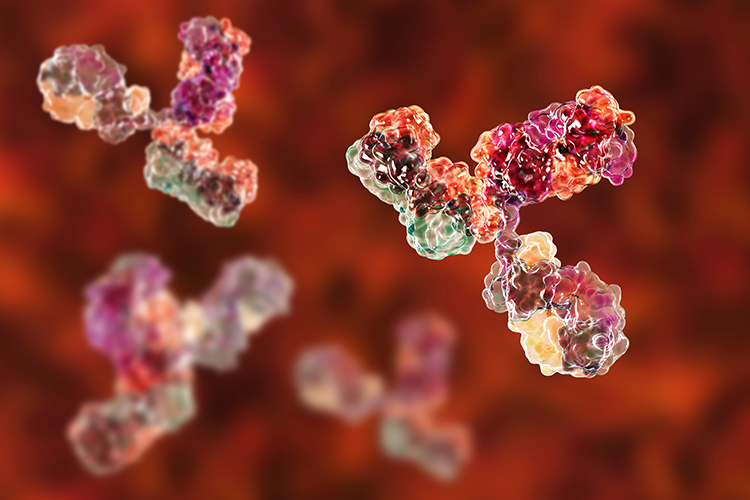
CHO manufacturing: scaling impossible heights
Antibody drugs have revolutionised several medical fields since breakthroughs in bioengineering in the 1980s enabled them to be manufactured monoclonally through Chinese hamster ovary (CHO) cell fermentation. Yet, so far they have not achieved their full theoretical potential. Almost all US Food and Drug Administration (FDA) approvals to date have been for diseases with lower worldwide prevalence; in particular, for oncology treatments and autoimmune disease in the developed world. They have not had a big impact on widespread health issues like the COVID-19 pandemic.
A key reason is the extreme lack of manufacturing capacity. According to industrial data provider BDO, global manufacturing capacity currently stands at approximately just 24,000kg/year.1 Even if 100 percent of that capacity were diverted to Regeneron’s anti-COVID-19 antibody cocktail – which is dosed at 1.2g per patient – that is just 20 million doses per year. Other estimates of underutilised CHO capacity are sometimes higher,2 but even assuming 10-times greater production, this is miniscule compared to a global population of eight billion.
Worse, because the serum half-life of injected antibody drugs is usually around one month, prophylactic use for diseases like COVID-19 would likely require monthly dosing, increasing demand to hundreds of millions of doses or more. Building a new CHO antibody plant costs upwards of $1 billion and two to three years, thus scaling up production to this degree is unfeasible. Furthermore, all antibody drugs currently on the market are delivered by injection, which requires rigorous purification protocols, further increasing the cost and scaling challenges.
Given this, it is not surprising that antibody drugs have not played a large role in the COVID-19 pandemic.
Rethinking antibody therapeutics from the fundamentals
An ideal drug is one that is not only effective and safe, but also widely available: a magic bullet. The breakthrough drugs of the mid-20th century offered precisely that; particularly small molecule antibiotics, which have revolutionised every aspect of medical practice. Yet when immunology pioneer Paul Ehrlich first coined the term “magic bullet” in the late 1800s, he was probably referring to antibodies, whose remarkable disease-fighting properties had been recently discovered. Making antibodies into drugs is therefore an old idea, but their impact on human health has so far failed to match that of antibiotics.
However, it is worth considering what might be done to expand their use to highly prevalent diseases like COVID-19.
Doing so means rethinking how they are delivered. Most infectious agents attack the body through its mucosal surfaces (SARS‑CoV-2 – the virus that causes COVID-19 – is unusual in that it attacks the mucosal tissues of both the respiratory tract and the gastrointestinal [GI] tract). This represents a challenge for injected antibodies because drug concentration at the site of infection is typically hundreds of times lower than in the blood. Even worse, the concentration of injected antibodies wanes rapidly over time as the body’s natural processes gradually break them down – a phenomenon referred to as the antibody’s ‘serum half-life’.
Maintaining a therapeutically meaningful concentration of injected antibody at the mucosal site of disease is therefore a major challenge. To overcome this, the few antibody drugs approved for use in infectious disease share a common feature: very large dose sizes. For example, the high dose in Regeneron’s Phase II COVID-19 clinical trial was 8 grams of pure antibody (115mg/kg); their Ebola antibody cocktail tips the scales at 150mg/kg.
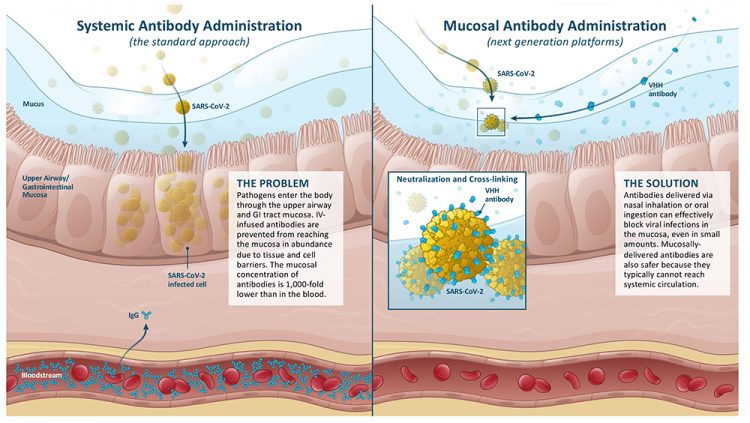
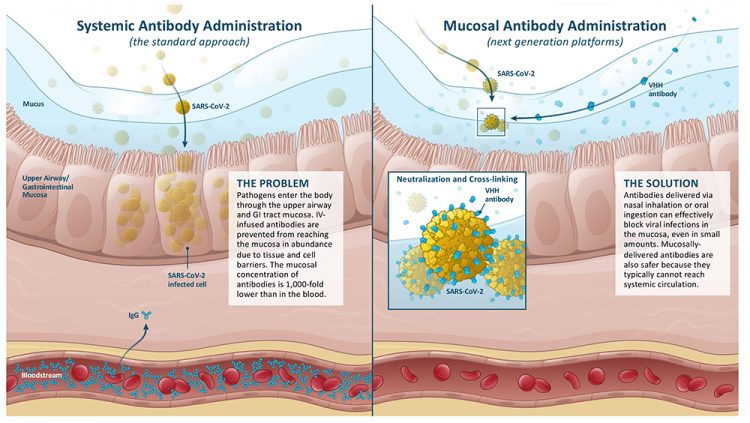
The role of mucosal tissue barrier function in enhancing or limiting the efficacy of injected versus topically applied antibody therapeutics. (Image credit: Lumen Bioscience and Cognition Studio).
Site-specific delivery – instead of injection – can address this challenge by ensuring the therapeutic antibodies are concentrated precisely where needed. In most cases, site-specific delivery should also enhance efficacy, since drug potency is often closely related to concentration. Indeed, most human clinical trials with orally delivered antibodies have reported encouraging results.3
However, solving the dosing problem in this way generates another challenge: antibodies are far more rapidly degraded and removed from mucosal tissues than in the relatively benign environment of the blood serum. The GI tract in particular is a one-way trip filled with proteases that are evolved to break down proteins. Thus, ensuring protection over time will mean frequent dosing, perhaps daily, driving up the overall quantities required.
Fortunately, there is a simple solution. The GI and respiratory tracts are constantly bathed in non-human proteins, not just by the wide variety of foods we eat and the dust we inhale, but also from the commensal bacteria teeming within us. Antibodies and other protein therapeutics can therefore safely be delivered topically to those tissues without the rigorous purification protocols required when antibodies are injected, dramatically enhancing scalability and reducing downstream costs.
The other half of the antibody-cost equation – that of growing them in the host organism, also called “upstream” production – can be solved with similar creativity. To avoid potentially dangerous immunogenicity issues, injected antibodies must hew closely to the structure of natural human antibodies, including mammalian glycosylation patterns. Mucosally delivered antibodies avoid these concerns because they are too large for intact absorption and remain confined to the immune-privileged environment of the GI and/or respiratory tract. This means that more efficient expression hosts can be used rather than finnicky mammalian cell systems like CHO.
Thinking beyond CHO
Several companies are now working to make mucosally delivered antibody drugs a commercial reality. The most established is Japanese biopharmaceutical company Astellas, which has been developing its Mucorice system for manufacturing therapeutic proteins in transgenic rice. Delivering monoclonal antibodies within whole rice kernels eliminates downstream processing costs entirely. Rice plants cannot make antibodies that perfectly copy those produced by mammalian cells in the way needed to safely inject them, but since they are intended for eating, not injection, this is fine.
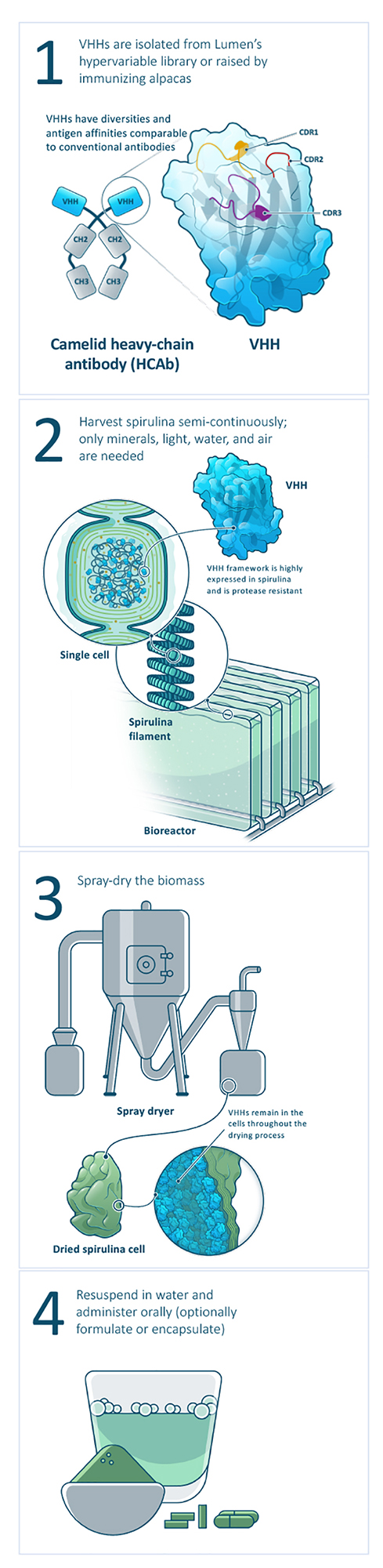
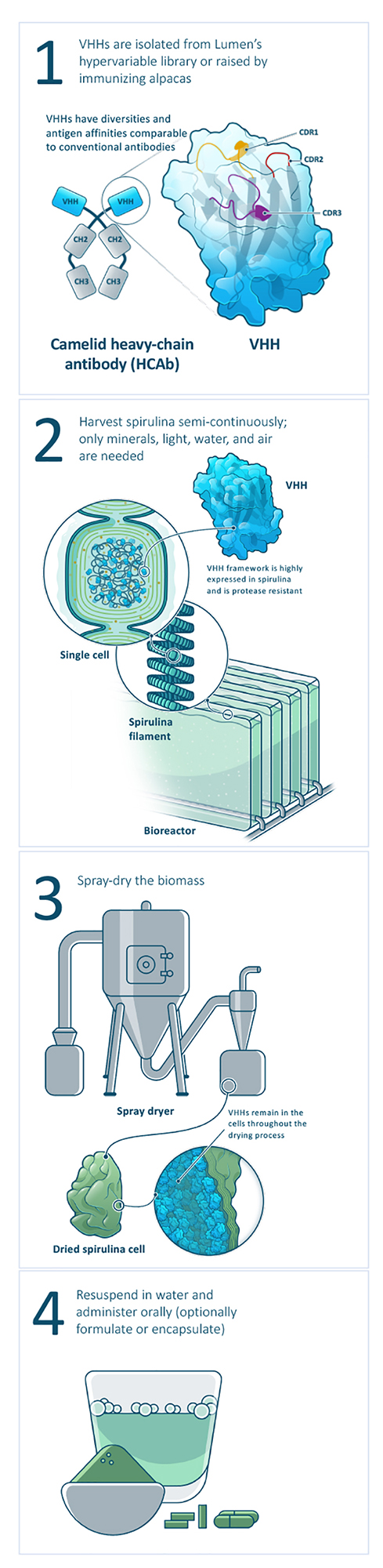
Lumen Bioscience’s fully integrated drug
development and GMP biomanufacturing
pipeline. (Image credit: Lumen Bioscience
and Cognition Studio).
The idea of delivering drugs like this – cheaply expressed and grown within a food crop – has, in the past, foundered on the challenge of achieving therapeutically meaningful expression levels and the high cost of stably bioengineering a food crop. However, Astellas has reported4 progress on the former using advanced RNAi techniques, and the CRISPR revolution holds obvious potential for redressing the latter. A third potential concern remains, however: grappling with consumer and regulator perceptions about sexually reproducing transgenic food crops.
Seattle-based Lumen Bioscience works with yet another food-based biomanufacturing system: spirulina, the only microbe farmed at commercial scale worldwide. Among photosynthetic microbes, spirulina specialises in making large amounts of soluble proteins. Its simple prokaryotic structure is amenable to accumulation of active protein in the cytoplasm at remarkably high levels, far beyond that reported in other food crop-based systems. Because spirulina is an asexually reproducing cyanobacterium amenable to indoor photobioreactor production, the risk of gene escape into the food chain can be effectively eliminated. Moreover, spirulina’s unicellular biology makes cell‑line establishment straightforward under ICH guidelines, bypassing the need for complex hybridisation and seed banking processes. Finally, cell line establishment and process engineering – major bottlenecks and cost drivers in the context of pandemic response – are simplified given the simple growth media and ease of bioengineering prokaryotic organisms like spirulina.
In a 2014 breakthrough, Lumen scientists developed the first methods for stably engineering new genes into the spirulina genome, a goal that had long frustrated researchers in the field.5 This bioengineering breakthrough allows drug developers to exploit an enormous amount of prior research into industrial-scale production of photosynthetic microbes.6 Initially focused on algae-derived biofuels – a vastly more difficult scaling challenge – the results translate readily to biopharmaceutical production. Most importantly, spirulina is already being produced at the kiloton quantities required for pandemic-scale impact, thus requiring no further scientific breakthroughs.
The cost of antibody production in CHO cells at the 2,000-4,000-litre scale is over $100 per gram, falling, by some estimates, to perhaps $20-40 per gram at the 75,000-litre scale.7 In contrast, conventionally grown spirulina powder retails for about two cents per gram of dried biomass, although Lumen’s current costs under current good manufacturing practice (cGMP) conditions are somewhat higher. Even so, current manufacturing costs are estimated at $1-2 per gram for a protein therapeutic delivered orally in whole cells and expressed at five percent of biomass. This is orders of magnitude lower than CHO and clearly scalable enough to contemplate making antibody therapeutics available globally for the first time.
Conclusions
Antibody therapeutics have transformed certain areas of medical practice over the last 30 years. However, unlike the biomedical breakthroughs of the mid-20th century, these drugs have so far primarily benefitted wealthy populations, an unfortunate consequence of the high cost of developing and making them in the traditional manner. For the same reasons, they have yet to make a global impact on ultra-prevalent diseases like COVID-19. Rethinking antibody drugs for the 21st century – and in particular re‑examining basic assumptions about how antibody drugs can be manufactured and delivered – is the key to helping antibody drugs realise their full potential for human health.
About the authors
Dr Craig Behnke is the Executive Vice President, Production and Development at Lumen Bioscience. After completing his PhD in Biochemistry from the University of Washington, he has applied his expertise in physical biochemistry and microbiology to problems ranging from small molecule drug discovery to biofuel production to biological therapeutic development. At Lumen, Dr Behnke brings his experience in biopharma R&D and large-scale cultivation of photosynthetic microbes to the challenge of repurposing spirulina for cGMP-grade biopharmaceutical production.
Lumen co-founder and CEO Brian Finrow trained at Harvard Law School and practiced law for nearly a decade with leading US biotechnology law firms, most recently Cooley LLP. After leaving private practice, he served in executive roles with successful biotechnology companies including AltAir Fuels, a leading producer of bio‑based jet fuel, and Adaptive Biotechnologies, a ground-breaking cancer diagnostics firm. In 2017, Finrow co-founded Lumen Bioscience, which is backed by leading venture capitalists and a wide range of public- and private-sector funding partners, including the Gates Foundation, BARDA/CARB-X, NIH/NIAID and the US Defense Health Agency. Lumen’s edible antibody cocktail directed at COVID-19, LMN-301, is scheduled to commence Phase II clinical trials in early 2022.
References
- Ecker DM, Crawford TJ, Seymour P. The Therapeutic Monoclonal Antibody Product Market. BioProcess International 2020 18(10)I.
- Sharma I, Wosińska, Kroetsch A, Sullivan H, McClellan M. COVID-19 Manufacturing for Monoclonal Antibodies. (Duke University Margolis Center for Health Policy) June 2020 (https://healthpolicy.duke.edu/sites/default/files/2020…
- Marcotte H, Hammarström L. Passive Immunization: Toward Magic Bullets. Mucosal Immunology 2015 Jan 1 (pp. 1403-1434). Academic Press. https://bioprocessintl.com/business/…
- Tokuhara D, Álvarez B, Mejima M, Hiroiwa T, Takahashi Y, Kurokawa S, Kuroda M, Oyama M, Kozuka-Hata H, Nochi T, Sagara H. Rice-based oral antibody fragment prophylaxis and therapy against rotavirus infection. The Journal of Clinical Investigation. 2013 Sep 3;123(9):3829-38.
- Takeushi R, Roberts J. “Targeted mutagenesis in spirulina” (PCT Global Patent Application) 2015. (https://patents.google.com/patent/WO2016040499A1)
- Benemann JR, Woertz I, Lundquist T. Autotrophic Microalgae Biomass Production: From Niche Markets to Commodities. Industrial Biotechnology 2018 Feb 1;14(1):3-10.
- Kelley B, Renshaw T, Kamarck M. Process and operations strategies to enable global access to antibody therapies. Biotechnology Progress. 2021 Mar 8:e3139.
Issue
Related topics
Antibodies, Biologics, Bioprocessing, Bioproduction, Drug Delivery Systems, Drug Manufacturing, Research & Development (R&D), Technology, Therapeutics, Vaccine Technology, Vaccines