The need for proteomic-based biomarkers in the drug development pipeline
Posted: 10 July 2012 |
Pharmaceutical companies are under increasing pressure to improve their efficiency and returns on drug discovery projects. This is a daunting task considering that the average drug costs approximately one billion US dollars to develop and takes around 12 years from initial discovery to reach the market1. In addition, approximately 70 per cent of drugs fail to recover their research and development costs and around 90 per cent fail to provide a satisfactory return on investment. Therefore, minimising risk is one of the most important aims in pharmaceutical discovery programs today.
There are now efforts to establish standard operating procedures to navigate through these problems and, at the same time, meet the regulatory demands. To facilitate this process, the regulatory health authorities have encour aged the incorporation of biomarkers into the drug discovery pipeline and the Food and Drug Administration (FDA) has called for efforts to modernise and standardise approaches for the delivery of more effective and safer drugs2.
Proteomics is the most applicable tech – nology for implementing biomarker app – roaches in drug discovery given that virtually all existing drug targets are proteins3. Proteomics is a systems approach for the global study of protein expression changes4.
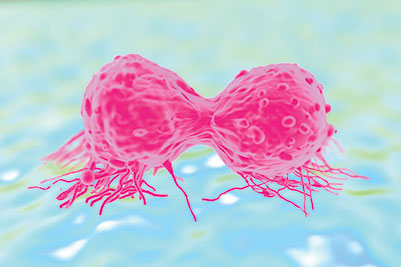
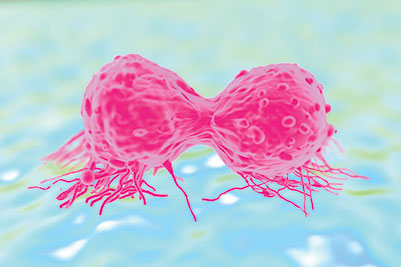
Pharmaceutical companies are under increasing pressure to improve their efficiency and returns on drug discovery projects. This is a daunting task considering that the average drug costs approximately one billion US dollars to develop and takes around 12 years from initial discovery to reach the market1. In addition, approximately 70 per cent of drugs fail to recover their research and development costs and around 90 per cent fail to provide a satisfactory return on investment. Therefore, minimising risk is one of the most important aims in pharmaceutical discovery programs today.
There are now efforts to establish standard operating procedures to navigate through these problems and, at the same time, meet the regulatory demands. To facilitate this process, the regulatory health authorities have encour aged the incorporation of biomarkers into the drug discovery pipeline and the Food and Drug Administration (FDA) has called for efforts to modernise and standardise approaches for the delivery of more effective and safer drugs2.
Proteomics is the most applicable tech – nology for implementing biomarker app – roaches in drug discovery given that virtually all existing drug targets are proteins3. Proteomics is a systems approach for the global study of protein expression changes4. It can provide information on gene function, disease processes and mechanisms of drug action at several stages in the drug discovery pipeline and pave the way for improved and faster implementation of drug discovery strategies. Ideally, clinically useful biomarkers should be readily accessible in body fluids such as blood serum or urine.
Application of proteomics in the discovery phase could positively impact on target identification, target validation, lead compound prioritisation and in vivo or ex vivo efficacy screening in preclinical models. Potential applications in the development phase include the generation of surrogate protein markers for drug efficacy and for the validation of animal models of human diseases5. Perhaps most importantly, proteomics can be used to identify protein signatures for diseases and use these signatures to derive new biochemical assays for disease diagnosis and to monitor drug efficacy in clinical trials. Proteomics can also be used to decipher interconnecting protein pathways of physiological processes6 which can help to improve our understanding of disease mech anisms and pave the way for improved rational drug design.
Proteomic methods
Two dimensional gel electrophoresis: the best known proteomics technology is two dimensional gel electrophoresis (2DGE)7. This method involves the separation of proteins according to isoelectric point (pI) using isoelectrofocusing (IEF), followed by apparent molecular weight (MW) separation using SDS-polyacrylamide gel electrophoresis. After 2DGE, gels can be stained using specific dyes for visualisation of protein spots, and the gel images can be scanned, protein spots detected and quantities determined using a 2DGE analysis software. Com – parisons across different gels can then be achieved by software matching of the same protein spots on different gel images, enabling statistical analyses to determine differences in protein expression. Thanks to the development of in-gel digestion protocols and further optimisations, 2DE spots can be manually or automatically extracted from 2DGE gels and indentified by mass spectrometry fingerprinting or sequencing (see below). Alternatively, fluorimetric prelabelling methods such as 2D difference gel electro phoresis (DIGE) can be used to increase the sensitivity and reproducibility of detection, although mass spectrometry-based methods are still required for identification8. The advantage of 2DGE is that it can generate information on intact proteins including any effects on post-translational modifications, such as phosphorylation or glycosylation changes. The disadvantage of 2DGE is the difficulty of analysing proteins of extreme physical properties, such as those with very high or low molecular weights or isoelectric points, and hydrophobic proteins such as integral membrane receptors.
Shotgun mass spectrometry: briefly, shotgun mass spectrometry is comprised of four stages: 1) digestion of hundreds of proteins in the proteome of interest to produce thousands of peptides 2) separation of peptides by high performance liquid chromatography (HPLC) 3) electrospray ionisation of the peptides and 4) time-of-flight mass spectrometry in which intact peptides and fragment ions are measured and quantitated9. The mass spectro meter measures the mass-to-charge (m/z) ratio of the ionised peptides and peptide fragments, and peptides can then be identified by com parison of the m/z values with those in a protein database which has been subjected to virtual proteolysis. The main advantage of this method is the ability to detect more difficult classes of proteins which are not detectable by 2DGE approaches. The disadvantages include the loss of intact protein information since proteins are digested prior to analysis.
Multiplex immunoassay profiling: this method uses distinct dye-coded microspheres for multiplex immunoassay analyses. The sample containing the proteome of interest is added to red/infrared dye-coded micro spheres containing covalently bound antibodies which target specific proteins. Next, secondary antibodies with a covalently-bound fluorescent label are added and this also binds to the protein of interest in a sandwich configuration. The mixtures are then passed through a flow cytometry-like instrument which uses lasers for identification of the coded antibody-microspheres and quantitation of the bound analytes. The advantage of this method is the high sensitivity, high throughput capacity and user friendly format. However, as all of the molecular profiling methods described above have different strengths and weaknesses, it is recommended that multiple methodologies are used for initial discovery. This would not only maximise proteome coverage, it would result in more comprehensive information on the relevant molecular pathways involved and thereby give increased confidence in the biomarker candidates identified.
Target validation
The majority of existing drug targets are components of a limited number of protein networks that have been therapeutically validated3. However, most of these networks are not fully elucidated in terms of the interacting protein components and relationship to other biological pathways. This raises the potential of mining these networks further for novel tractable drug targets. Identification of specific proteins that are pivotal in the disease process is typically the first stage of target validation. This can sometimes be achieved by manipulating the expression of proteins in these networks using gain or loss of function techniques in an attempt to reverse the disease phenotype10. Increasing the function of the protein of interest could be achieved by agonist-type small molecules or over-expression technologies. Alternatively, the protein could be knocked down functionally using antagonistic small molecules, ribozymes, small interfering RNAs or conditional genetic approaches. In each case, a proteomic signature could be obtained for monitoring the resulting phenotype. Such approaches would give increased confidence that small molecules under drug development would have a similar effect.
For validation and prioritisation of targets, it is important to establish the molecular context or interaction pathways associated with potential drug targets. This involves under – standing the disease at a functional level and confirming the therapeutic concept in animal models and clinical proof of principle app – roaches. Such approaches could be used to validate animal models of the disease to ensure the relevant pathways are sufficiently similar to warrant their use in drug discovery. Proteomics and expression profiling studies can provide this information by identifying components of cellular networks that could be targeted for possible therapeutic intervention. Techniques such as the Tandem Affinity Purification (TAP) method have been used to elucidate protein networks11. Such subsets of proteins are termed interactomes and could incorporate signalling partners with a given target protein and thereby lead to identification of novel drug targets.
Lead optimisation
Many compounds fail in the later stages of drug development because of unexpected toxicity or poor efficacy12. Therefore, a greater under – standing of drug properties is needed at an earlier stage in the development pipeline. Proteomics can generate expression signatures from cells or tissues treated with a compound for target identification and validation, and for delineating the mechanism of action of compounds. Proteomic signatures can also be used in the identification and optimisation of lead compounds by correlations with efficacy or a specific toxicity. For example, monitoring the effects of developmental compounds on protein expression patterns in the appropriate models might provide an early prediction of efficacy or toxicity13. Compounds which induce the same signature of protein expression changes are presumed to share the same mode of action and toxicity effects. The ultimate aim is to use changes in proteomic patterns to understand how drugs exert their effects at the molecular level.
Drug toxicology studies
Successful drugs should be potent, specific and orally bioavailable with good pharma – cokinetics and low toxicity. Ideally, compounds lacking one or more of these traits should be identified during the early stages of the drug discovery pipeline so that only the most promising compounds are taken through to clinical trials. Toxicities often do not become apparent until the preclinical or clinical development stages of drug discovery when compound testing is initiated in animal models or humans. In some cases, toxicities may not even be detected until widespread distribution of the drug to the general public14. The reasons for this can be complex and are sometimes attributable to the metabolism of the parent compound to produce toxic metabolites or to poor clearance of toxic components. Proteomics can accelerate development of the best lead compounds by implementation of screening methods for toxicity based signatures.
Clinical studies
Clinical proteomic applications include early detection of disease using the proteomic signatures in body-fluids as a complement to any histopathology or other measures carried out to specifically target the affected biological pathway in patients. Once a particular signature for efficacy has been established, this can be applied in a high throughput format such as screening with multiplexed immunoassays to help in the identification of compounds with optimum profiles. Also, prognostic biomarkers can be used to predict drug efficacy in patient populations15,16 and to identify which patients are likely to benefit from specific drugs. This could help to pave the way for development of more individualised therapies17. The ultimate application of proteomics in drug discovery would be to identify biomarkers in a readily accessible body fluid such as serum, and which can be correlated with the initiation of efficacy or severity of toxicity. Such biomarker signatures could also be used as surrogate markers to help predict the response of individ – uals to treatment and allow tailoring of the therapy to achieve optimal efficacy without toxic side effects. Likewise, this approach could be used to facilitate identification of patient classes who will respond favourably to the drug in clinical trials. Therefore, the use of proteomic profiling technologies will provide a better understanding of drug efficacy, metabolism and toxicity, reduce costs for drug manufacturers and result in safer drugs for patients.
We recently developed the first multiplex immunoassay biomarker test for schizophrenia18,19. This test comprised of 51 immunoassays which allowed reproducible identification of schizophrenia patients compared to healthy control subjects with high sensitivity and specificity. Validation of this test consisted of developing a linear support vector machine decision rule and testing the performance of this using cross-validation20. This resulted in readjustment of the panel and algorithm to a smaller set of 40 robust assays, along with a simple procedure for maintenance and recalibration across future measurement changes associated with different reagent lots. The resulting decision rule delivered a sensitive and specific prediction for the presence of schizophrenia in subjects compared to matched controls, with a receiver operating characteristicarea under the curve of 88 per cent. This multiplexed assay system was launched in 2010 as a CLIA-approved test to aid diagnosis of schizophrenia.
Conclusions
We have described the emerging use of proteomic-based profiling as an enabling platform for use in all aspects of the drug discovery process (Figure 4 opposite). This is critical as current diagnostic procedures and strategies for developing novel medicines are in need of a paradigm change21. The regulatory health authorities now consider the incorpor – ation of biomarkers into clinical platforms to be of critical importance for the future drug discovery and have called for efforts to modernise methods, tools and techniques to achieve this goal. Given the complex nature and low abundance of many proteomic biomarkers, this will most likely require the development of more sensitive methods and a massive integration of tech nologies. However, there is now reason to be optimistic that such proteomic-based methods will continue to emerge given the recent advances in this area.
References
1. Hughes, J.P., Rees, S., Kalindjian, S.B., Philpott, K.L. (2011). Principles of early drug discovery. Br J Pharmacol 162, 1239-1249
2. Ovens, J. (2006) Funding for accelerating drug development initiative critical. Nat Rev Drug Discov 5: p271
3. Imming, P., Sinning, C. and Meyer, A. (2006) Drugs, their targets and the nature and number of drug targets. Nat Rev Drug Discov 5, 821-834
4. Wilkins, M.R., Williams, K.L., Appel, R.D. and Hochstrasser, D.F. Editors. Proteome Research: New Frontiers in Functional Genomics (Principles and Practice). Springer; illustrated edition, 1997. ISBN: 3540627537
5. Sarnyai et al. (2011) Behavioral and molecular biomarkers in translational animal models for neuropsychiatric disorders. Int Rev Neurobiol 101, 203-238
6. Castellana, N. and Bafna, V. (2010) Proteogenomics to discover the full coding content of genomes: a computational perspective. J Proteomics 73, 2124-2135
7. O’Farrell, P.H. (1975) High resolution two-dimensional electrophoresis of proteins. J Biol Chem 250, 4007-4021
8. Unlü, M., Morgan, M.E., Minden, J.S. (1997) Difference gel electrophoresis: a single gel method for detecting changes in protein extracts. Electrophoresis 18, 2071-2077
9. Aebersold, R., Mann, M. (2003) Mass spectrometrybased proteomics. Nature. 422, 198-207
10. Sioud, M. (2007) Main approaches to target discovery and validation. Methods Mol Biol 360, 1-12
11. Forler et al. (2003) An efficient protein complex purification method for functional proteomics in higher eukaryotes. Nat Biotechnol 21, 89-92
12. Amacher, D.E. (2010) The discovery and development of proteomic safety biomarkers for the detection of drug-induced liver toxicity. Toxicol Appl Pharmacol 245, 134-142
13. Meneses-Lorente et al. (2006) Identification of early proteomic markers for hepatic steatosis. Chem Res Toxicol 19, 986-998
14. Gale, E.A. (2001) Lessons from the glitazones: A story of drug development. Lancet 357, 1870-1875
15. Killestein, J., Polman, C.H. (2011) Determinants of interferon β efficacy in patients with multiple sclerosis. Nat Rev Neurol 7, 221-228
16. Gerger, A., Labonte, M., Lenz, H.J. (2011) Molecular predictors of response to antiangiogenesis therapies. Cancer J 17, 134-141
17. Flood, D.G., Marek, G.J. and Williams, M. (2011) Developing predictive CSF biomarkers-A challenge critical to success in Alzheimer’s disease and neuropsychiatric translational medicine. Biochem Pharmacol 81, 1422-1434
18. Schwarz et al. (2010) Validation of a blood-based laboratory test to aid in the confirmation of a diagnosis of schizophrenia. Biomark Insights 5: pp39-47, 2010
19. Schwarz et al. (2011) Identification of a biological signature for schizophrenia in serum. Mol Psychiatry 2011. Apr 12. [Epub ahead of print]
20. Izmailov et al. (2011) Algorithm development for diagnostic biomarker assays. Int Rev Neurobiol 101, 279-98
21. Poste, G. (2011) Bring on the biomarkers. Nature 469, 156-157
About the authors
Dr Paul C. Guest is Head of Proteomics at the Cambridge Centre for Neuro – psychiatric Research at Cambridge University. He also has 10 years of experience in heading the biomarker facility at the Merck Sharp & Dohme Neuroscience Research Centre and eight years of experience working in the field of metabolic disorders. He has published numerous articles in the fields of neuro – science, stem cells, metabolic diseases and biomarkers in high impact journals.
Professor Sabine Bahn is a practising clinician, leading research scientist and Director of the Cambridge Centre for Neuropsychiatric Research at Cambridge University. Her main research interests are to understand the molecular basis of neuropsychiatric disorders, with a focus on schizophrenia and bipolar disorder. Professor Bahn has published numerous articles. In 2005, she co-founded Psynova Neurotech Ltd., which, together with Rules Based Medicine has launched the first blood test aiding in the early diagnosis of schizophrenia.
Issue
Related topics
Biomarkers, Drug Discovery, Drug toxicology studies, Proteomics