Raman spectroscopy: a useful tool in the fight against COVID-19
Posted: 14 December 2020 | Nikki Withers (European Pharmaceutical Review) | 1 comment
Raman spectroscopy has numerous applications in the pharmaceutical industry, ranging from verification of raw materials to quality control of products. This article explores how researchers are using the technique to aid our understanding of SARS-CoV-2, potentially improving detection speed and methods of analysis.
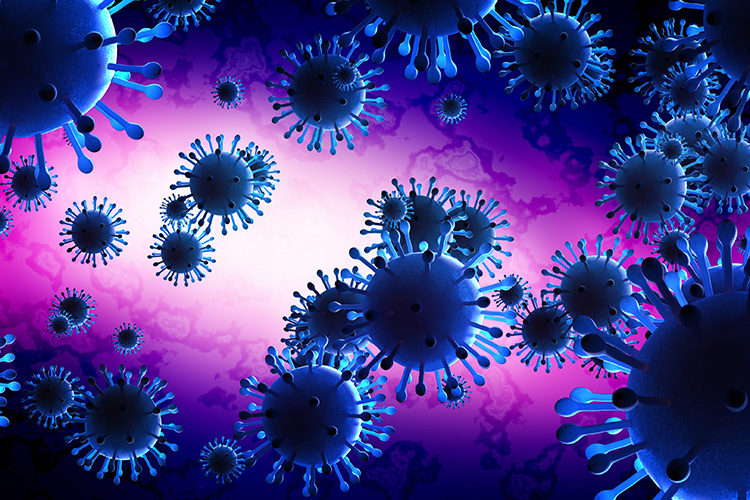
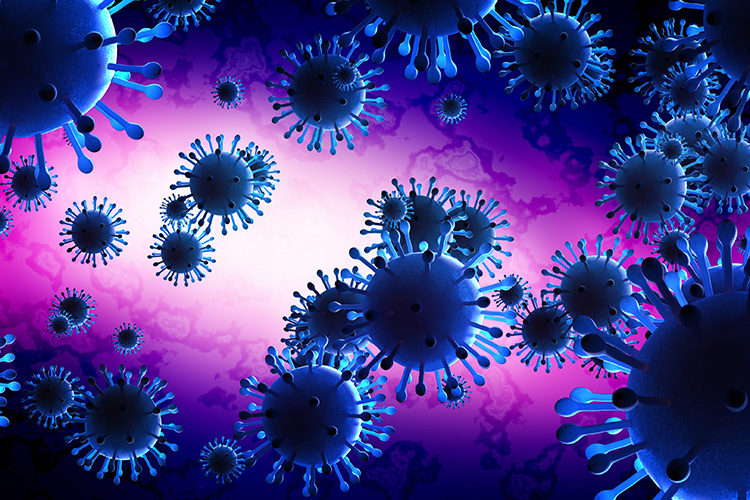
RAMAN SPECTROSCOPY is a vibrational spectroscopy technique that has many applications in the pharmaceutical industry. It is used to detect vibrational, rotational and other states in a molecular system and can be used to probe the chemical composition of materials. Variations of the technique – for example, surface-enhanced Raman scattering (SERS) or tip-enhanced Raman scattering (TERS) – offer further insights for low concentration or trace analysis. Since the first reported case of Severe Acute Respiratory Syndrome Coronavirus 2 (SARS-CoV-2) in December 2019, researchers around the world have worked tirelessly to understand the virus. Several groups have been using Raman to assist in this global effort, applying it to enhance environmental detection, speed up testing and to explore a saliva fingerprint for those infected with COVID-19. Here, we explore these studies in more detail.
Environmental detection
Researchers from Tsinghua University, the Research Institute for Environmental Innovation and the Chinese Academy of Sciences have published a paper1 showing how they used nano-rod silver SERS substrates to help detect COVID-19 in the environment.
SERS can be used to interrogate trace materials by exploiting the electromagnetic field enhancement resulting from the excitation of localised surface plasmon resonances at nanostructured metallic surfaces, such as gold or silver. “We developed an assay using SERS coupled with multivariate analysis to diagnose SARS‑CoV-2 in an ultra-fast manner, without any pre‑treatment, eg, RNA extraction,” explained the researchers.
Their research was based on the knowledge that the SARS-CoV-2 Spike (S) glycoprotein consists of two subunits – S1 and S2 – and that the S1 subunit contains a receptor-binding domain (RBD) that specifically recognises the human receptor angiotensin-converting enzyme 2 (ACE2) for cell entry. “Such recognition and binding might alter the structure of ACE2 and lead to changes in the Raman spectra,” they predicted. “Additionally, the binding specificity allows ACE2 to act as an anchor to capture SARS-CoV-2 from human or environmental specimens for interrogation.”
Using their silver-nanorod SERS array functionalised with ACE2, designated ACE2@SN-SERS, the researchers obtained strong SERS signals of ACE2 at 1,032, 1,051, 1,089, 1,189, 1,447 and 1,527cm-1. They also found that recognition and binding of the RBD of the SARS-CoV-2 S protein on the SERS assay significantly quenched the spectral intensities of most peaks and exhibited a shift from 1,189 to 1,182cm-1.
Furthermore, when they performed on-site tests of 17 water samples with a portable Raman spectrometer, the team, led by Dr Dayi Zhang from Tsinghua University, reported satisfactory performance in interrogating the presence of SARS-CoV-2 in environmental specimens. “On-site tests… proved its accuracy and easy operation for spot diagnosis of SARS-CoV-2 to evaluate disinfection performance, explore viral survival in environmental media, assess viral decay in wastewater treatment plants and track SARS-CoV-2 in pipe networks,” they said.
The researchers concluded that, compared with real-time reverse transcription quantitative polymerase chain reaction (RT-qPCR) and serological enzyme-linked immunosorbent assays (ELISA), which are also used to detect SARS-CoV-2, their ACE2@SN-SERS assay had three significant advantages. “Firstly, the ACE2@SN-SERS assay does not rely on RNA extraction or immune biomarkers, simplifying the sample preparation procedure and shortening the measurement time. Secondly, the S protein is more stable than RNA as the biomarker for SARS-CoV-2, suggesting it is a more stable and sensitive assay particularly for environmental specimens. Lastly, we have proved that portable Raman spectrometers can provide satisfactory signals with the ACE2@SN‑SERS assay and allow rapid interrogation of SARS-CoV-2 on site.” The researchers said their work is promising for the development of fast and accurate screening tools, which could help to fight the COVID-19 pandemic.
Rapid testing
Earlier this year a second group of scientists, from the Northern Arizona University (NAU) in the US, received a $200,000 grant from the National Science Foundation’s Rapid Response Research (RAPID) funding programme supporting virus-related research.2 Their aim was to develop SERS substrates as a testing aid for fast COVID-19 detection. “The project team will use non-traditional techniques to detect virus in infected patients,” explained NAU professor Miguel José Yacamán, a physicist and materials scientist in the Center for Materials Interfaces in Research and Applications (¡MIRA!). “We will develop an alternative method based on recent advances in physics related to the interaction of light with matter.”
During the pandemic, one of the most challenging aspects for researchers has been the lack of testing to detect and trace infections. Furthermore, the limitations in quantities and quality of test kits have resulted in uncertainties about public health policies. “Testing is necessary not only for diagnosis but also for tracking to control the pandemic,” the researchers noted. They explained that one of the practical challenges of increasing the number of available test kits is difficulty in producing the chemicals needed for the test. The most used test, RT-qPCR, is expensive, has a long turnaround time and, although it is highly sensitive, produces many false negatives and is based on biochemical methods.
Their present work utilises Raman spectroscopy in its single molecule (SM)‑SERS modality. The method is based on amplification of the inelastically scattered light by localised surface plasmons on metal nanoparticles. The main goal is to establish a Raman signature of the S glycoprotein of the SARS-CoV-2 virus that can be used to develop a detection test. “The ability of SM-SERS to detect as little as one molecule of protein will enable healthcare professionals to detect infection early and follow up with patients who recover from the illness,” said Yacamán.
In his previous work, Yacamán used SERS to detect glycoproteins and sialic acid as a method of testing for breast cancer, which is now in the final approval stage for commercial use. These tests, based on the analysis of sialylated glycoproteins, were shown to have high sensitivity, specificity and a low percentage of false negatives. “In the case of the SARS-CoV-2 virus, it was a natural extension to apply the same techniques,” he said, noting that it required the expertise of his colleagues at NAU’s Pathogen and Microbiome Institute (PMI) who were growing the SARS-CoV-2 virus in their labs.
The project team will develop a new type of plasmonic nanoparticle combined with ionic liquids that will improve the nanoparticle delivery to solutions containing the virus. A second interest will be studying the fine peak structure, which is related to morphological changes in the proteins. “If successful, our research will be the first step in developing a method based on physics that will be fast and inexpensive, with high sensitivity and specificity and a low percentage of false negatives,” said Yacamán. “This test will be a much more precise and reliable method to detect infections.”
He concluded: “It is conceivable that once we have developed this test, portable Raman equipment can be used widely in many different populations; for instance, in rural or remote communities or in point-of-care stations in schools, factories, community centres and so on, in addition to traditional testing sites. Once widespread testing is under way, further analysis of the SM-SERS data will help scientists understand changes on the virus proteins and help develop antiviral drugs.”
COVID-19 saliva fingerprint
A third group of researchers is using Raman spectroscopy on saliva samples to characterise and validate the salivary Raman fingerprint of COVID-19.3 “One of the first infection sites of SARS-CoV-2 is the oral cavity where the virus is able to bind and penetrate through the ACE2 receptors present on the epithelial cells of the salivary glands,” explained the researchers. “Thus, a high concentration of virus particles could be found in saliva in the preliminary phases of the infection.” They added that Raman spectroscopy is a non-invasive, fast and label-free vibrational technique, which can provide information regarding presence, concentration, environment, modifications and interactions of all the biochemical species present in a specific biofluid, including saliva.
Using Raman spectroscopy, the researchers will analyse saliva collected from 40 healthy individuals, 40 patients infected with COVID-19 and 40 individuals with a past infection of COVID-19. “Before the analysis, a drop of saliva (3μl) will be deposited on aluminium foil and dried overnight. The aluminium foil is fundamental to achieve the SERS, increasing in this way the saliva Raman signal,” they said.
The study, which commenced on 1 June 2020, had six primary outcome measures:
- Identification and characterisation of a new COVID-19 salivary signature through Raman spectroscopy. This signature will then be able to discriminate individuals with a current or past infection.
- Evaluation of the spectral differences between the experimental groups. The Raman data collected from the experimental groups will be compared and interpolated with the huge number of Raman databases on biofluids present in literature. This procedure will provide a determination of the principal biochemical species involved in the differences between the experimental groups (eg, viral structural proteins and lipids, cytokines, inflammatory molecules, damaged biomolecules).
- Determination of the classification model through multivariate analysis. The Raman database will be processed through principal component and linear discriminant analysis. The consecutive leave‑one‑out cross-validation will provide a primary discrimination model that can assign each spectra to one of the experimental groups.
- Correlation with the clinical data. Raman data related to individuals with a current or past infection by SARS-CoV-2 will be correlated with the clinical data, validating the methodology. The principal correlation will be carried out between the severity of the respiratory infection and the time between the first SARS-CoV-2 positive test and the last negative SARS-CoV-2 test.
- Test of the methodology. The classification model will be continuously questioned and trained using new potential patients and adding new clinical parameters as “sub-groups” for the complete discrimination and prediction of the pathological state.
- Portable Raman as point-of-care. The characterised and implemented classification model will be translated to a portable Raman device equipped with a laser emitting at 785nm and with a spectral resolution comparable with that of the bench Raman. This station will be firstly tested with patients presenting to the hospital and then applied continuously, implementing the classification model with new Raman spectra and clinical data. In this way, they will be able to implement the accuracy, sensitivity, precision and specificity of the model.
The study is due to be completed by 1 June 2021. The principal aim of the project is to create a classification model that is able to: discriminate COVID-19 current and past infection; identify the principal biological molecules altered in saliva during the infection; predict the clinical course of newly diagnosed COVID-19 patients; and translate and apply the classification model to a portable Raman device for the test at a point-of-care.
References
- Zhang D, Zhang X, Ma R, Deng S, Wang X, Zhang X, et al. Ultra-fast and onsite interrogation of Severe Acute Respiratory Syndrome Coronavirus 2 (SARS-CoV-2) in environmental specimens via surface enhanced Raman scattering (SERS). 2020.
- NSF Award Search: Award#2030488 – RAPID: Development of a New Test for SARS-CoV-2 using Single Molecule Surface Enhanced Raman Spectroscopy [Internet]. Nsf.gov. 2020 [cited 8 December 2020]. Available from: https://www.nsf.gov/awardsearch/showAward?AWD_ID=2030488&HistoricalAwards=false
- The Salivary Raman COVID-19 Fingerprint – Full Text View – ClinicalTrials.gov [Internet]. Clinicaltrials.gov. 2020 [cited 8 December 2020]. Available from: https://clinicaltrials.gov/ct2/show/NCT04583306
Issue
Related topics
Environmental Monitoring, Raman Spectroscopy, Screening, Viruses
Related organisations
Chinese Academy of Sciences, Northern Arizona University (NAU), the Research Institute for Environmental Innovation, Thermo Fisher Scientific, Tsinghua University, WITec
very good and informative article.