Approval of regulatory starting materials
Posted: 27 October 2020 | Dave Elder (David P Elder Consultancy) | No comments yet
There are significant interpretational differences between the European Medicines Agency (EMA) and US Food and Drug Administration (FDA)’s guidance on active pharmaceutical ingredient (API) starting materials. In this article, Dave Elder explores the ambiguity in guidance from both agencies and addresses why industry struggles to identify and justify starting materials that are likely to garner regulatory approval in both these territories.
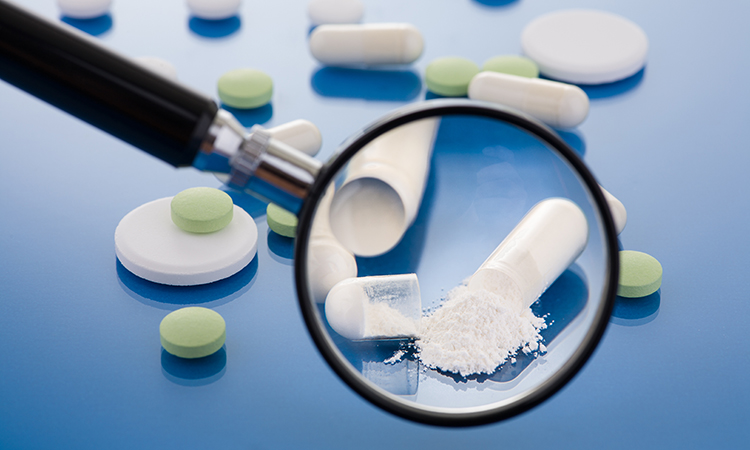
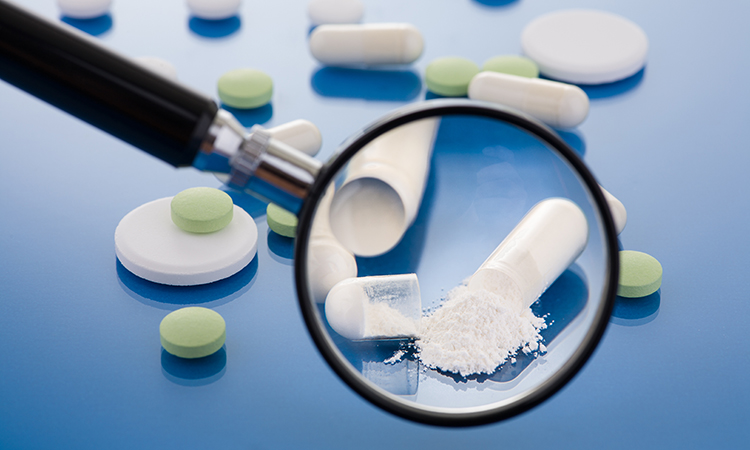
ONE OF the biggest challenges faced during API development is the “designation and justification” of the API registered starting materials (RSMs); ie, those stages in the API synthesis where current good manufacturing practice (cGMP) philosophies and practices are first introduced.1 Indeed, the FDA’s cautionary statement from the first guidance in 1987 still holds true: “What constitutes the ‘starting material’ may not always be obvious.”2
ICH Q73 defined an RSM as: “A raw material, intermediate or an API that is used in the production of an API and that is incorporated as a significant structural fragment into the structure of the API. An API starting material can be an article of commerce, a material purchased from one or more suppliers under contract or commercial agreement or produced in-house. API starting materials are normally of defined chemical properties and structure.” However, the guidance failed to provide insight into the “designation and justification” of the API RSM.1
ICH Q11: development and manufacture of drug substances
Subsequently, ICH Q11 was introduced during 2011 to harmonise the criteria for selection and definitions of the RSMs.4 ICH Q11 covers the development and manufacture of APIs and provides guidance on these materials. Each branch of a linear or convergent API manufacturing process begins with one or more RSM. An RSM should be a material with well-characterised chemical structure and properties, as such, non-isolated intermediates produced via telescoped chemistry are usually not considered appropriate RSMs.
ICH Q11 was introduced during 2011 to harmonise the criteria for selection and definitions of the RSMs”
The definition of “significant structural fragment” in this guidance is intended to differentiate RSMs from “reagents, solvents or other raw materials”. However, in retrospect the terminology was often misconstrued and misinterpreted by industry to support the use of structurally complex RSMs; whereas the intent was the reverse and regulators wanted industry to designate significantly simpler molecules than the API to be used as RSMs.5,6 Commonly available materials used to create salts, for example methane sulfonic acid, esters (eg, ethanol) or other simple derivatives should be considered reagents.
The guidance indicates that the connectivity between overall risk and number of stages from the introduction of an RSM to the completion of the manufacturing procedure is a combination of two main factors. The first relates to the physical properties of the API; which are defined during the final crystallisation stage and any subsequent size reduction processes, eg, milling, micronising, etc. The second factor relates to the formation, fate and purge of impurities, particularly mutagenic impurities (MIs).7 Impurities that are introduced or generated in the early stages of the manufacturing process typically have significantly more opportunity for removal (or purging) during subsequent downstream purification operations, for example extraction, distillation, washing or isolation/crystallisation of isolated intermediates, than those impurities generated late in the manufacturing process. Consequently, late-forming impurities are more likely to be carried over into the final API.
The prime considerations for any analytical method are that it exhibits appropriate specificity and sensitivity”
Although ICH Q114 aimed to harmonise practises intended to identify appropriate RSMs, many companies still interpret this guidance differently. Equally worryingly, the “target of regulators is not aligned across regions and continuously reinterpreted”.1 In the EU, during the early years of the ICH Q11 guidance (2010-2011), “inconsistency of approaches” was seen from reviewers across the region. During this time period, two-thirds of all Certificate of Suitability (CEP) dossier applicants were requested to redefine their RSMs based on a failure to demonstrate “an appropriate control strategy”.1 The European Directorate for the Quality of Medicines (EDQM) issued additional guidance to their assessors during 2012 to try and harmonise the interpretation of the ICH Q11 guidance.4 This additional guidance, while mainly aligned with ICH Q11, argued that the number of steps between the RSM and the API can be related to the overall control strategy; therefore, there was an expectation that more supporting information was required for late-stage RSMs to demonstrate that the applicant has appropriate process understanding to demonstrate that the quality of the subsequent API can be adequately controlled. Thus propinquity (the number of GMP steps) was an important consideration within the EU region and remains so.1 Indeed, the EMA will often go beyond reduction in structural complexity and a robust impurity control strategy to request additional stages between the proposed RSM and the API.
The increased frequency of disagreements between regulatory agencies and applicants has led to both the EMA5 and FDA6 issuing question and answer (Q&A) supplements for ICH Q11. Both agencies indicated that “applicants should consider all the ICH Q11 general principles… rather than just choosing a few of the general principles”.5,6 They highlighted the importance of MI control when identifying potential RSMs. Non-MIs should be controlled to ICH Q3A(R2)8 identification thresholds; in contrast, MIs should be controlled to 30 percent of the toxicology-based threshold defined in ICHM7(R1).9 However, they did add the caveat that for drugs for advanced cancer indications (as per ICH S910) or APIs that are themselves mutagenic, ICH Q3A(R2) criteria8 would apply. Both agencies also stated that there should be “multiple steps” between a non-commercially available RSM and the API, unless the structural complexity of the proposed RSM is low, in which case it can be assessed in a similar fashion to a commercially available RSM.5,6
The key challenges still remain: suppliers are always trying to maximise yield, minimise the environmental impact of the process and reduce cost whilst still aligning to the designated quality specification; applicants will continually seek to ensure there is always more than one supplier capable of manufacturing the RSM to the designated quality criteria as part of business continuity strategies; and regulators will always seek to limit applicants choice of supplier and the ability of applicants to change RSM suppliers with the objective of ensuring consistent quality.11
Analytical strategies
Therefore, in order to “future proof” the designated RSM method from the impact of potential upstream chemistry changes leading to new impurities, it is always prudent to develop a fully-validated analytical method (as per ICH Q2(R1)15) for the RSM, with sufficient specificity and resolving power to detect any new impurities that could arise from any future process changes. Method validation is recommended by both the EMA5 and FDA.6 Indeed, full validation of the methods is the typical practice within industry, ie, in 92 percent of all cases, with the remainder of methods qualified.16 The typical RSM method is ICH Q3A(R2) aligned8 from a sensitivity perspective, with most companies having a reporting threshold of 0.1 percent.16 Companies typically employ tighter analytical controls, including impurity fate and purge studies, for RSMs introduced late in the process, ie, closer to the API, due to “limited impurity purging opportunities”.16 Industry also has a strong focus on potential mutagenic impurity (PMI) control strategies. Most companies will perform a full mutagenic risk assessment on the upstream RSM processes and develop appropriately sensitive methodology to follow the downstream fate of any PMIs.16
Risk-based strategies
Given the ambiguity facing companies when trying to “designate and justify” RSMs,1 some companies have tried to predict the risk and uncertainty using model-based approaches, typically focusing on RSM complexity, sourcing, process robustness and impurity controls.16 Wigman et al.17 focused on four main parameters: propinquity, impurity purging power, complexity and stability, where a high risk (>10) would indicate that the likelihood of regulatory approval was decreased. However, some commentators have observed that the weight given to impurity control parameters (and particularly MIs) does not accurately reflect the importance that this factor plays in gaining regulatory approvals. Although the purging factor was developed primarily to assess the removal of reactive, ie, MIs or PMIs from API syntheses,7 the basic physicochemical attributes that are important in purging are equally germane to normal impurities, ie, ICH Q3A(R2)8 designated impurities.
In the majority of cases, industry tries to mitigate this risk by initiating contingency strategies”
Reizman et al. performed a retrospective analysis of 34 small molecule RSMs where they had requested scientific advice from the FDA, 12 of which were also reviewed by the EMA; ie, a third were assessed by both agencies.18 They noted a reduction in regulatory endorsement over time. The team achieved 92 percent agreement over the time period 2000-2005, but this dropped to 71 percent over the period 2006-2015. However, they also noted that their figures were likely to be a “conservatively high approximation”.18 Using the methodology of Wigman et al.17 Reizman and colleagues demonstrated 91 percent success with scores of ≤12, whereas they only saw 58 percent approval for scores of ≥13. An important qualification to their approach was the increased focus on MIs or PMIs. They developed the model further and identified two important parameters linked with successful endorsement of RSMs: (i) complexity score and (ii) impurity risk score. The team reported that their success metrics were impressively high for both endorsement, ie, 100 percent or non-endorsement, ie, 87.5 percent when complexity and impurity risk scores were either met, or not met, respectively.18 However, the concern is that, elegant though the model is, it fails to take into account the cultural differences between the FDA (pragmatic, risk-based) and the EMA (more conservative in nature).These tools help development teams to “recognise risks early”, allowing them to appropriately focus valuable process development resources.17 Reizman et al. used this approach to assess four alternative routes to a very complex API, which was currently synthesised using 16 chemical transformations. They were able to significantly reduce the number of stages of chemistry down to seven, without meaningfully increasing the likelihood of regulatory push-back on their proposed RSMs.18 The team similarly used this risk-based model to decide between two alternative commercial synthetic routes for a development candidate, based on a comparative assessment of the likelihood that the designated RSMs would be approved.
Conclusions
Although both the EMA5 and FDA6 have issued supplementary guidance (in terms of Q&A) to ICH Q11 guidelines,4 industry still struggles to identify and justify RSMs that are likely to garner regulatory approval in both territories. In the majority of cases, industry tries to mitigate this risk by initiating contingency strategies whereby the stages immediately preceding the initially designated RSM, ie, n-1 or n-2, are also manufactured to GMP standards and if they fail to persuade the relevant agency of the merits of their initial RSM designation(s), then this alternative mitigation strategy can be initiated. However, this is an inefficient and expensive commercial strategy and it would be much better to be able to predict the likelihood of success.
Reizman et al.18 have claimed that their risk-based approach helps to answer the thorny question of what is “acceptable simplicity” with respect to RSMs. Both Reizman et al.18 and Wigman et al.17 have used risk-based modelling and identified that the molecular complexity of the proposed RSM is the most significant factor impacting on regulatory acceptance. The other related factor is control of impurities (particularly PMIs) in the RSM and the two factors are intrinsically linked as increased complexity may be indicative of more intricate chemistry that will require greater impurity control. Unfortunately, Reizman et al.’s risk-based strategy is probably too US-centric, ie, only about one third of the small molecules assessed were evaluated by both the FDA and EMA,18 and thus fails to adequately model cultural differences. As such, the two agencies still do not always agree on the choice of designated RSM.
About the author
Dave Elder has nearly 40 years of service within the pharmaceutical industry at Sterling, Syntext and GlaxoSmithKline. He is now an independent GMC consultant. He is a visiting professor at King’s College, London and is a member of the British Pharmacopoeia. He is a member of the Joint Pharmaceutical Analysis Group (JPAG) and the Analytical Division Council of the Royal Society of Chemistry.
References
- Faul MM, Kiesman WF, SmulkowskiM, Pfeiffer S, et al. Part 1: A review and perspective of the regulatory guidance to support designation and justification of API starting material. Org. Proc. Res. Dev., 18, 2014, 587-593.
- FDA. Guideline for submitting supporting documentation in drug applications for the manufacture of drug substance. Center for drug evaluation and research (CDER), 1987.
- ICH Q7A. Good manufacturing practices for active pharmaceutical ingredients. Step 5, CPMP/ICH/4106/00, November 2000.
- ICH Q11. Development and Manufacture of Drug Substances. Step 5. EMA/CHMP/ICH/425213/2011, November 2012.
- ICH Q11. Development and Manufacture of Drug Substances. Questions and Answers. Step 5. EMA/CHMP/ICH/809509, 01 September 2017.
- Q11. Development and Manufacture of Drug Substances. Questions and Answers. Guidance for Industry. February 2018.
- Teasdale A, Elder DP, Chang S-J, Wang S et al. Risk assessment of genotoxic impurities (GTIs) in new chemical entities-strategies to demonstrate control.Org. Proc. Res. Dev., 17(2), 2013, 221-230.
- ICH Q3A(R2). Impurities in new drug substances, Step 5. CPMP/ICH/2737/99. October 2006.
- ICHM7(R1). Guideline M7(R1) on assessment and control of DNA reactive (mutagenic) impurities in pharmaceuticals to limit potential carcinogenic risk, Step 5. EMA/CHMP/83812/2013. February 2018.
- ICH S9. Guideline S9 on nonclinical evaluation for anticancer pharmaceuticals. Step 5. EMA/CHMP/ICH/646107, May 2010.
- Seevers RH, Klutz WF.Regulatory strategy for starting material designation.Pharm. Outsourcing. Jan/Feb2010, 11, 1.
- Holm R, Elder DP. Analytical advances in pharmaceutical impurity profiling. Eur. J. Pharm. Sci., 87, 2016, 118-135
- Li X, Stoll DR, Carr PW. A simple and accurate equation for peak capacity estimation in two-dimensional liquid chromatography. Anal. Sci., 81(2), 2009, 845-850.
- Faul MM, Busacca CA, Eriksson MC, Hicks F, et al. Part 2: Designation and justification of API starting materials: Current practices across member companies of the IQ consortium.Org. Proc. Res. Dev., 18, 2014, 594-600.
- ICH Q2(R1). Validation of analytical procedures: Text and Methodology, Step 5. CPMP/ICH/38/95. June 1995.
- Faul MM, Argentine MD, Egan M, Eriksson MC, et al. Part 3: Designation and justification of API starting materials: Proposed framework for alignment from an industry perspective. Org. Proc. Res. Dev., 19, 2015, 915-924.
- Wigman L, Schulte Oestrich R, Hildbrand S, Iwamura H, et al. A new risk assessment tool for regulatory starting material evaluation. Amer. Pharm. Rev., 16 March 2017.
- Reizman BJ, Burt JL, Frank SA, Argentine MD, et al. Data-driven prediction of risk in drug substance starting materials. Org. Proc. Res. Dev., 23, 2019, 1429-1441.