Solid-state NMR spectroscopy of drug substances and drug products
Posted: 29 October 2020 | Eric Munson (Purdue University) | 1 comment
Solid-state NMR spectroscopy is an effective technique for the analysis of both crystalline and amorphous pharmaceuticals, both in the drug substance and drug product. In this article, Eric Munson provides an overview of the technique and how it applies to pharmaceutical analysis.
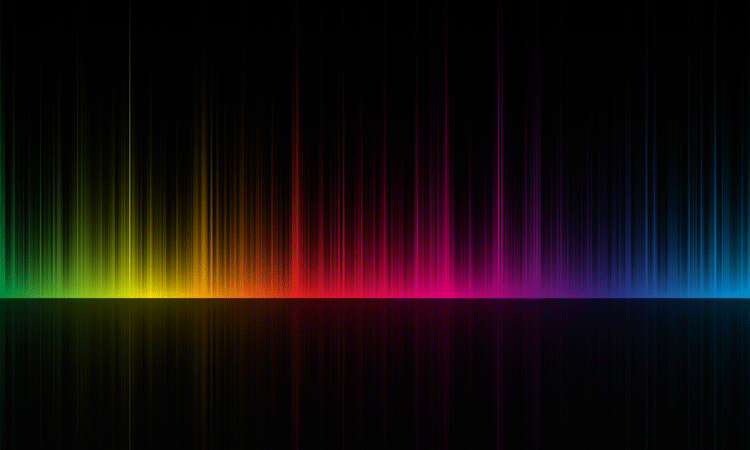
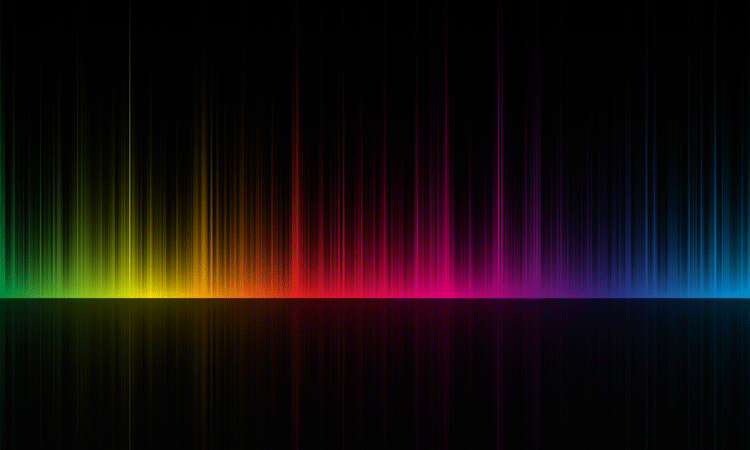
CHARACTERISATION of drug substances and products is critical in the pharmaceutical industry. Solid-state NMR spectroscopy (SSNMR) is an excellent analytical technique used to characterise the solid forms of a drug substance, identify different crystalline structures, monitor form conversion during active pharmaceutical ingredient (API) scale up, detect low amounts of other forms (eg, crystalline in amorphous), measure relaxation times for prediction of physicochemical stability and guard intellectual property. Similarly, drug product is uniquely suited to characterisation by SSNMR because excipients typically do not significantly interfere with the analysis, even at low levels of drug substance in the drug product.
The need for characterisation of drug forms
The physical state of a drug substance and its potential interactions with components in a drug product play a critical role in drug delivery. For example, different solid forms, eg, polymorphs, solvates and amorphous forms, can dramatically impact the amount of drug available to the body (bioavailability) and the ability to successfully make a drug product (manufacturability) by altering physicochemical properties such as solubility and morphology. In many cases, the ability to identify the most stable crystalline form in an API within a drug product is critical to prevent conversion from one crystalline form to another, which could impact the performance of the drug product. Most pharmaceutical companies conduct a series of screening tests to determine both the most stable crystalline form, as well as any potential polymorphic or solvated forms of the API. In many cases, it can be quite difficult to isolate the individual forms that may be found in the API and the drug product.
Why SSNMR spectroscopy?
SSNMR spectroscopy is a particularly powerful technique for the investigation of multiple crystalline forms in both an API and drug product. The benefits of SSNMR spectroscopy include:
- it is complementary to powder X-ray diffraction for identifying different crystalline forms
- it is the only commonly-used analytical technique that can quantify the amount of each form without the need for a standard
- it can detect and quantify the amount of crystalline and amorphous content in an API
- it can identify the amount of crystalline and amorphous content for each component in a formulated product
- multiple nuclei can be analysed, providing several unique spectra for each form
- relaxation times can be used to identify peaks for different crystalline forms, as each relaxation time for a form is usually unique
- relaxation times can be used to selectively enhance or remove different forms in a mixture of components, which can be useful for form identification and showing intellectual property infringement
- selective labeling of nuclei such as 13C and 15N can be used to enhance certain signals when sensitivity is a challenge, as well as avoiding peak overlap
- relaxation times can be used to identify particle size and predict chemical degradation rates, especially in formulated products.
Detecting polymorphs using SSNMR spectroscopy
SSNMR spectroscopy has several advantages for studying polymorphism in pharmaceuticals, including the fact that there are typically several NMR active nuclei that can be studied and each can provide unique information about the sample. The most commonly studied nuclei for identifying multiple crystalline forms in both a drug substance and drug product are 13C and 19F, with lesser studied nuclei including 15N, 31P, 23Na, 35Cl and others.
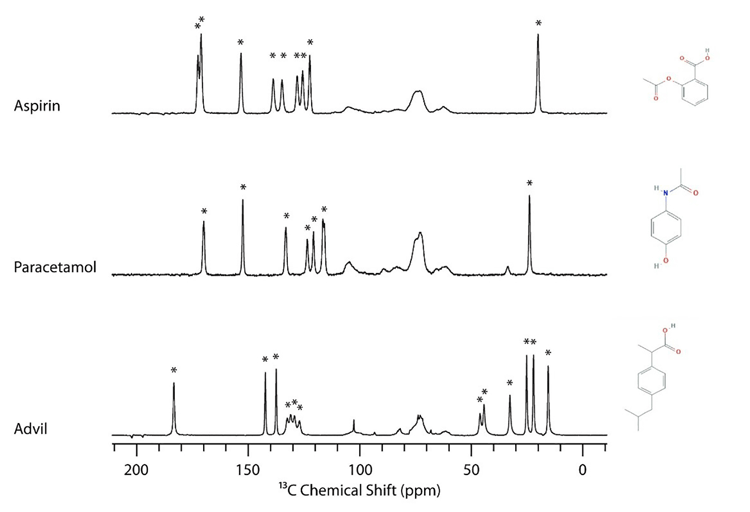
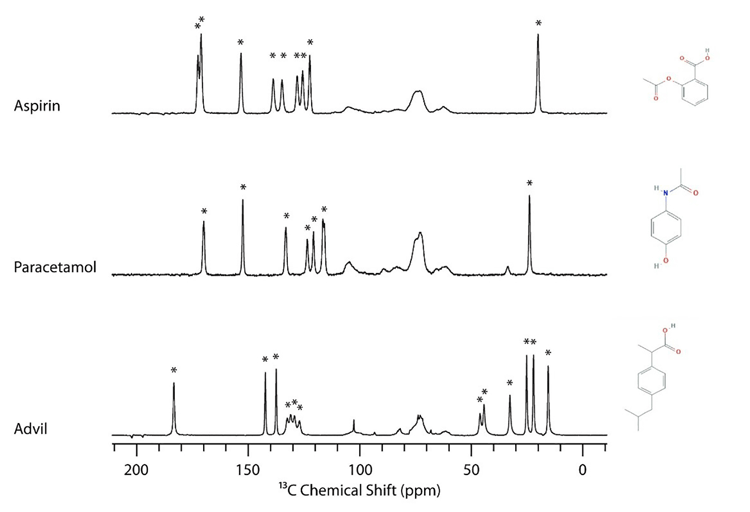
Figure 1: 13C solid-state NMR (SSNMR) spectra of aspirin, paracetamol and ibuprofen (Advil).
Active pharmaceutical ingredient (API) peaks are indicated by a star (*).
For 13C, there are typically between 10 to 30 peaks in the NMR spectrum for an averagesize pharmaceutical compound that can be used to identify the particular crystalline form being studied. One advantage of 13C SSNMR compared to other analytical techniques such as powder X-ray diffraction is that the peaks for the excipients tend to be between 60 to 110ppm, whereas drugs will tend to have peaks between 0 to 50ppm and 110 to 200ppm. This is highlighted in Figure 1, which shows the 13C SSNMR spectra of aspirin, acetaminophen (paracetamol) and ibuprofen in their respective formulations. The API peaks are indicated by a star. This example shows that many drug peaks do not overlap with excipients and can be used to identify the particular crystalline form.
In 19F SSNMR, the only peaks in the spectrum are due to drug substance, because no excipients contain fluorine. Figure 2 shows the 19F spectra of polymorphs A and B of triamcinolone. Although the bottom spectrum is five percent w/w in a formulation, the only obvious difference between this and the API spectrum (middle) is the scaling factor used to make both spectra a similar intensity.
SSNMR spectroscopy can also provide information about the number of crystallographically inequivalent sites in the crystal lattice. In particular, for 13C SSNMR the presence of two peaks for a specific carbon usually indicates that there are two different conformations/ arrangements of molecules in the asymmetric unit. Likewise, there could be three or four peaks for each carbon, corresponding to that number of molecules in the asymmetric unit. In Figure 2, there are two peaks in the 19F spectrum for each polymorphic form due to two crystallographically inequivalent molecules in the crystal lattice.
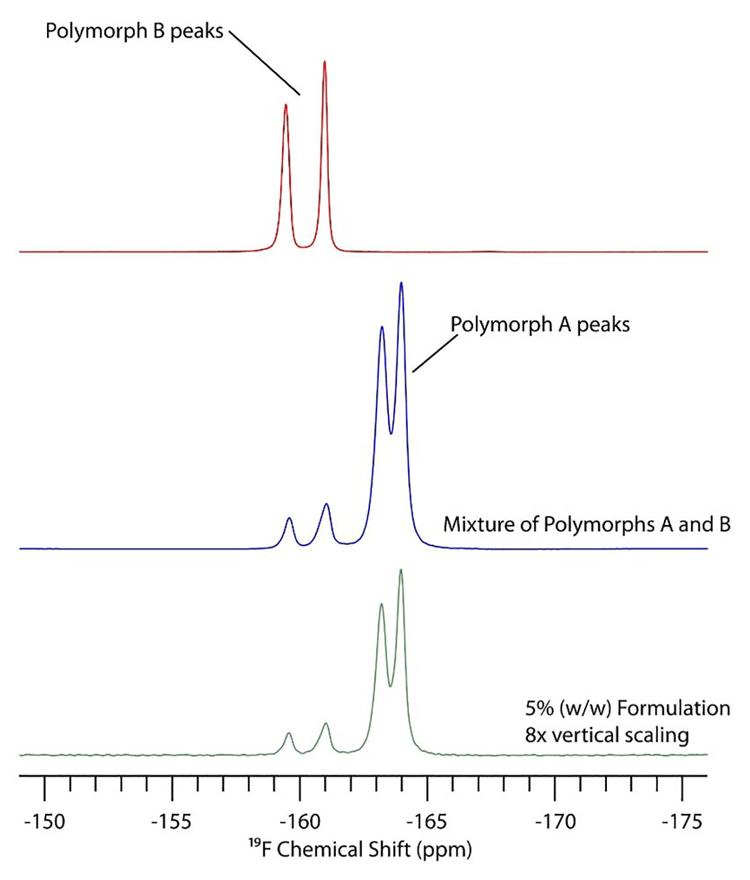
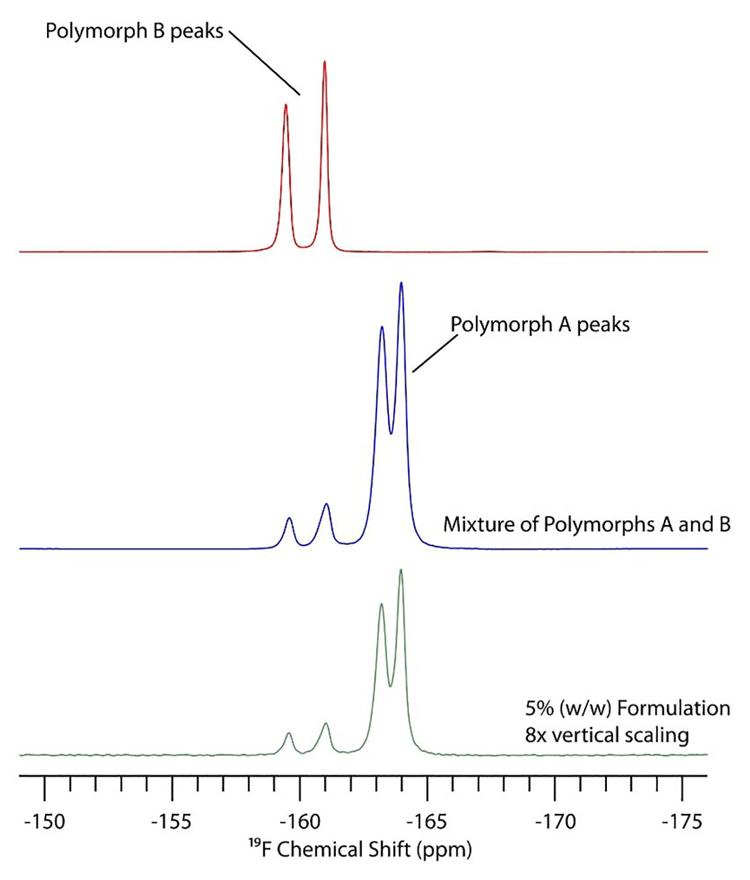
Figure 2: 19F solid-state NMR (SSNMR) spectra of triamcinolone API polymorph B (red), a mixture of API polymorph A and B (blue) and the same mixture in a formulation with five percent w/w API.
Quantitation and limit of detection in SSNMR spectroscopy of polymorphs
One of the benefits of SSNMR spectroscopy is that, unlike most other analytical techniques, it is an inherently quantitative technique. In NMR spectroscopy, the response function of each nucleus in the molecule in theory should be the same. This means that the integration of the peaks in an NMR spectrum reflect the amount of each form that is present in the sample. This assumes that the spectra were acquired using quantitative conditions. In most other analytical techniques, a calibration curve has to be prepared, because the response function of the instrument, whether it be the extinction coefficient in optical spectroscopy, such as UV-Vis spectroscopy, or the peak intensity in a powder X-ray diffraction pattern, which could depend upon factors such as preferred orientation or particle size affects, is unknown. In most analytical methodologies, including NMR spectroscopy, the signal-to-noise ratio of 3:1 is often used to determine the limit of detection (LOD) and a signal-to-noise ratio of 10:1 is often used to determine the limit of quantification (LOQ).
LOD and LOQ for polymorphs for SSNMR spectroscopy can vary significantly depending upon line widths, relaxation times and compound molecular weight. In general, LOD of 0.1-1 percent are easily achievable for drug substances that have molecular weights of around 400g/mol, line widths of 0.5ppm or less and 1H T1 relaxation times of 10 seconds or less, which are typical of many drug substances. Low LOD and LOQ are often limited in drug substances by the ability to resolve the different form peaks in the spectrum. If the forms have different relaxation properties, then experiments that can reduce or remove one component selectively can be used to observe the desired component. The detection limits are absolute in that low-dose drug products, eg one to five percent of drug substance by weight in a drug product, still have a drug substance detection limit of 0.1-1 percent, but being able to resolve the different forms is less of a problem.
Amorphous drugs and amorphous solid dispersions
Amorphous solid dispersions (ASD) are being used more frequently in drug products to increase drug bioavailability. An ASD can increase bioavailability several fold, which reduces the pill burden on the patient and may even make a drug candidate viable. One of the challenges of an ASD is that the API is in a metastable state, ie, it would prefer to be in the crystalline state. Since even a small amount of crystalline material could result in decreased bioavailability, regulatory agencies often require monitoring of the drug product for crystallinity. SSNMR spectroscopy is an excellent technique for determining crystallinity in a drug product. For 13C SSNMR, the amorphous peaks are often about an order of magnitude broader than the crystalline peaks. The LOD and LOQ for the crystalline component in an ASD are similar to that of polymorphs, except that the amorphous signal is usually substantially broader than the crystalline signal, making overlap less of an issue compared to a mixture of two crystalline forms. The LOD for an amorphous API is usually substantially higher, often by an order of magnitude compared to the crystalline component, due to line widths that are significantly broader than the crystalline component.
Emerging technologies for SSNMR analysis of pharmaceuticals
Conclusion
SSNMR spectroscopy is an outstanding technique for the analysis of both crystalline and amorphous pharmaceuticals both in the drug substance and drug product. It is an inherently quantitative technique that can selectively detect the API separately from the excipients. Multiple nuclei can be studied, where each nucleus can provide unique information about the sample. For example, selective labelling of 13C nuclei can be used to both increase sensitivity and isolate signals from a particular functional group, while the 19F SSNMR spectrum only contains signals from the API and not the excipients. Moreover, relaxation filters and multidimensional experiments can be used to isolate components and monitor dynamics, respectively. The detection limits for polymorphs are often superior to other analytical techniques and are usually unaffected by the presence of excipients in drug products.
About the author
Eric Munson is currently the Dane O. Kildsig Chair and Head of the Department of Industrial and Physical Pharmacy at Purdue University. His research programme is focused on the characterisation of pharmaceutical solids using a variety of analytical techniques, with an emphasis on solidstate NMR spectroscopy. He received his BA degree from Augustana College in Sioux Falls and his PhD from Texas A&M University. He was also a postdoctoral fellow at the University of California, Berkeley. After working in the Chemistry Department at the University of Minnesota he moved to the Pharmaceutical Chemistry Department at the University of Kansas in 2001, the Pharmaceutical Sciences Department at the University of Kentucky in 2010 and he took up his current role at Purdue University in 2018. Eric is a coinventor on three patents and has published more than 100 research, review and book chapters.
Disclosure
Eric is partial owner of Kansas Analytical Services, a company that provides solidstate NMR services to the pharmaceutical industry. Figures 1 and 2 were prepared using data from Kansas Analytical Services.
References
- Alia Hassan et al., Sensitivity Boosts by the CPMAS CryoProbe for Challenging Biological Assemblies, J Magn Reson 2020, 311: 106680
- Qing Zhe Ni et al., In Situ Characterization of Pharmaceutical Formulations by Dynamic Nuclear Polarization Enhanced MAS NMR, J Phys Chem B, 2017, 121: 8132
- Dirk Stueber and Stefan Jehle, Quantitative Component Analysis of Solid Mixtures by Analyzing Time Domain 1H and 19F T1 Saturation Recovery Curves (qSRC), J Pharm Sci 2017, 106: 1828
Issue
Related topics
Active Pharmaceutical Ingredient (API), Analytical techniques, QA/QC, Spectroscopy
Nice Article, In my company NMR is newly installed can you provide more info on NMR spectroscopy.