Peracetic acid in the study of rhIL-11 methionine oxidation
Posted: 19 June 2008 | Nicholas W. Warne, Rebecca L. Koval and Thomas J. Crowley, Drug Product Development Group, Wyeth Biopharma, Andover | No comments yet
Recombinant human interleukin-eleven (rhIL-11) is a pleiotropic cytokine which stimulates stem cell proliferation and affects multiple cell types1. The protein has been demonstrated to provide clinical benefit in platelet restoration2 and Crohn’s disease3. rhIL-11 is a highly basic protein that is rich in arginine, leucine and proline residues, lacks disulfide bonds, is not glycosylated and is not homologous with other known hematopoietic growth factors4. In the development of stable dosage forms of rhIL-11, we have examined the protein’s stability toward methionine oxidation.
Recombinant human interleukin-eleven (rhIL-11) is a pleiotropic cytokine which stimulates stem cell proliferation and affects multiple cell types1. The protein has been demonstrated to provide clinical benefit in platelet restoration2 and Crohn’s disease3. rhIL-11 is a highly basic protein that is rich in arginine, leucine and proline residues, lacks disulfide bonds, is not glycosylated and is not homologous with other known hematopoietic growth factors4. In the development of stable dosage forms of rhIL-11, we have examined the protein’s stability toward methionine oxidation.
Recombinant human interleukin-eleven (rhIL-11) is a pleiotropic cytokine which stimulates stem cell proliferation and affects multiple cell types1. The protein has been demonstrated to provide clinical benefit in platelet restoration2 and Crohn’s disease3. rhIL-11 is a highly basic protein that is rich in arginine, leucine and proline residues, lacks disulfide bonds, is not glycosylated and is not homologous with other known hematopoietic growth factors4. In the development of stable dosage forms of rhIL-11, we have examined the protein’s stability toward methionine oxidation.
Methionine oxidation has been reported as an important instability in several proteins, including human interleukin-5 and human growth hormone5-8. To examine this potential instability, we have utilised reagents that will specifically oxidise methionine residues in an attempt to simulate and predict the oxidation behavior of rhIL-11 on long-term stability. Further, chemical oxidation of rhIL-11 will allow us to understand the ability of various assays to demonstrate stability-indicating properties. The primary sequence of rhIL-11 is provided in Figure 1. The sequence shows two methionine residues (Met58 and Met122).
Chemical oxidation of proteins has been studied using several reagents. Specifically, several cytokines and growth factors have been examined. Hydrogen peroxide has been used to oxidise bovine growth hormone, human interleukin-2, human somatrotropin and human chorionic somatomammotropin9-12. One criticism of hydrogen peroxide, however, is that additional reactive functional groups such as thioethers, indoles, sulfhydryls, disulfides, imidazoles and phenols have been noted13. Chloramine-T has been used to oxidise human interleukin-6 and equine growth hormone14,15. Chloramine-T, however, has been demonstrated to oxidise tyrosine and histidine residues16. Human relaxin has been studied by three approaches: hydrogen peroxide, light stress and ascorbic acid/Cu+2 oxidation17-19. Human interferon-γ has been studied using t-butyl hydroperoxide20. We have chosen to study the oxidising potential of peracetic acid as a specific methionine-oxidising reagent.
In Chen et al21, sulconazole, an anti-mycotic drug susceptible to oxidation, was examined by reaction with peracetic acid and hydrogen peroxide. The authors noted that peracetic acid is 105 times more reactive at pH 7 than hydrogen peroxide. Similiarly, Lambert et al22 demonstrated that peracetic acid is 1.2 x 104 to 2 x 106 times more effective in the disinfection of Ps. aeruginosa and Staph. aureus, respectively. Because of this strong reactivity, we designed our experiments to oxidise the methionine residues of rhIL-11 using sub-stoichiometric levels of peracetic acid to determine which of the two methionines were more susceptible to oxidation.
Materials
rhIL-11 was produced at Wyeth BioPharma. All experiments were performed at a concentration of 0.5 mg/mL (26 μM) in 10 mM NaH2PO4, pH 7.0. Oxidations were performed using peracetic acid (Sigma-Aldrich) or hydrogen peroxide (Sigma). Incubations were performed in polypropylene HPLC vials in a Waters WISP autoinjector or a Waters Alliance 2690 Separations Module at room temperature.
Methods
Oxidising reagents were diluted in 10 mM NaH2PO4, pH 7.0 to an appropriate concentration (10-50 μM for peracetic acid, 20-100 mM for hydrogen peroxide). Oxidising reagent was combined with buffer and rhIL-11 solution to generate a protein concentration of 0.5 mg/mL (26 μM). Since two methionines exist per protein molecule, the methionine concentration was 52 mM. After gentle inversion (1-mL in a polypropylene cryo-vial) 200 μL aliquots were distributed to polypropylene HPLC vials for the Waters WISP autoinjector/separations module. Every 16 minutes, 20 μL of sample was removed from the tube and injected onto the HPLC column. Using this approach, the oxidation reaction occurred within the autoinjector instrument.
Reversed-phase HPLC was performed using a Waters HPLC system (Waters 600E pump, WISP autoinjector, 490E multi-wavelength detector or a Waters Alliance 2690 Separations Module with a 996 PDA detector and Empower software). The reversed-phase HPLC column was a 4.6 x 50 mm Poros R1/H (Perseptive Biosystems) using a mobile phase of 0.1% TFA (A) and 90% acetonitrile in 0.1% TFA (B) and a linear gradient at 3 mL/min.
Loss of rhIL-11 was determined by comparing the remaining area of the rhIL-11 peak versus the initial peak area. The data was fitted to a single exponential decay equation to determine the rate constant of the loss of rhIL-11 using Grafit v2.0. Similarly, the rate of generation of oxidised Met58 and Met122 was examined and the first order rate constant determined. Double oxidised rhIL-11 (Met58 and Met122) was also measured and used in the calculations for methionine oxidation. Sample chromatographs of rhIL-11 before and after oxidation are presented in Figure 2.
The stability of rhIL-11 as an aqueous dosage form was monitored over a 24-month period at 2-8ºC. Samples were incubated at 5 mg/mL in a 2-mL molded glass vial using a West 4416/50 13 mm Teflon coated serum stopper. The buffer system was 10 mM NaH2PO4, 300 mM glycine, pH 7.0. The solution was filtered using a 0.2 um PVDF membrane (Durapore®), then the vials were filled under a laminar flow biosafety cabinet. At selected timepoints, samples were examined to determine the levels of methionine oxidised rhIL-11 using reversed-phase HPLC. The RP-HPLC method for the stability study differed from the RP-HPLC method for the chemical oxidation study in that the gradient and loading conditions were lengthened for best resolution of the various species induced on stability.
Results
Generation of methionine-oxidised rhIL-11 on long-term stability
rhIL-11 was examined by various stability-indicating assays to determine its structural integrity as a function of time and temperature. One of the critical assays is reversed-phase HPLC for monitoring the oxidation of methionine residues Met58 and Met122 (see Figure 2 for an example). Data on rhIL-11, formulated at 5 mg/mL in aqueous solution and stored at 2-8°C, is presented in Figure 3. The data shows firstly that a higher level of Met58 is oxidised in the starting material than Met122, and secondly that Met58 oxidised more rapidly than Met122 under these conditions. Comparison of the rates of methionine oxidation show that Met58 oxidises at 0.12% / month (r2 = 0.67) and Met122 oxidises at 0.066% / month (r2 = 0.92). Comparison of these rates shows that Met58 oxidised approximately two times more rapidly than Met122 under these storage conditions.
Chemical oxidation of methionine residues
Initial experiments were performed to determine the optimal conditions for the chemical oxidation of rhIL-11 using hydrogen peroxide and peracetic acid. Figures 4a and 4b show the generation of oxidised Met58 and Met122 using 60 mM hydrogen peroxide and 35 μM peracetic acid, respectively. The data in Figure 4a (60 mM hydrogen peroxide) show a gradual increase in the levels of Met58 and Met122 oxidation up to 120 minutes. While a slight plateau effect is observed with the generation of oxidised Met58, no such plateau is observed with Met122. Figure 4b (35 μM peracetic acid) shows a rapid oxidation of both methionine residues, however a distinct plateau effect is observed. This plateau is presumably due to exhaustion of the peracetic acid. In comparing the two figures, Met58 clearly oxidises more rapidly than Met122.
Comparison of the rates of rhIL-11 oxidation as a function of oxidant concentration, is shown in Figure 5. The data clearly demonstrates that the peracetic acid induced oxidation of methionine is approximately 1000 times more rapid than the hydrogen peroxide induced oxidation. Further, the peracetic acid oxidation experiments were all performed at sub-stoichiometric concentrations and appeared to reach a plateau dependent on the concentration of the oxidant. Hydrogen peroxide oxidation reached a plateau for Met58 oxidation, presumably due to complete oxidation of the amino acid, however oxidation did not reach a plateau for Met122, indicating that oxidation continued through the last timepoint (120 minutes).
Examination of the rates of peracetic acid induced oxidation shows that as the concentration of peracetic acid is increased, the rates of Met58 and Met122 oxidation also increase. Further, increases in peracetic acid concentration lead to increased levels of oxidation. This is shown in Table 1. The data shows that increases in peracetic acid concentration lead to different
levels of oxidation for Met58 and Met122. Specifically, as the oxidant concentration increases, the ratio between the two methionine residues changes from 5.1 to 1.5. Completeness of methionine oxidation will therefore influence the observed stoichiometry of methionine oxidation. Summation of the Met58 and Met122 levels, as seen in Figure 6, shows a stoichiometric relationship between the amount of oxidant used in the experiment and the amount of methionine oxidation observed within rhIL-11. The slight difference between the expected and observed levels of oxidation are probably due to potential variability in the concentration of the peracetic acid starting solution.
Discussion
Incubation of aqueous rhIL-11 demonstrates that Met58 oxidises twice as fast as Met122. Similarly, chemical oxidation of rhIL-11 with hydrogen peroxide and peracetic acid shows that Met58 oxidises faster than Met122. The preference for oxidation of Met58 is presumably due to its orientation on the outside of the protein, which could increase its solvent accessability. This is consistent with the model proposed by Czupryn et al23,24 in which alanine scanning mutagenesis and protein modification was utilised to study the protein fold of rhIL-11. Methionine modification using iodoacetic acid24 demonstrated that Met58 was more readily modified than Met122. This was demonstrated using a comparable RP-HPLC method as well as an Asp-N peptide map method. In our studies, oxidation of Asp-N digested rhIL-11 with peracetic acid demonstrated that Met58 and Met122 were oxidised at comparable rates (data not shown). This data, combined with that of Czupryn et al24, suggests that protein conformation is responsible for the selective preference for Met58 oxidation.
The consistency between long-term stability and the chemical modification results demonstrates the utility of chemical oxidation experiments, in that they can be predictive of oxidation susceptability on long-term storage. Further, oxidation of rhIL-11 with hydrogen peroxide and peracetic acid is useful in generating oxidised protein, which could be utilised as materials for developing analytical methods, such as reversed-phase HPLC, which will detect and monitor the stability of rhIL-11. Such an approach was taken in developing the stability-indicating assay described in this report. Examination of the oxidised forms of rhIL-11 show that the oxidised protein elutes with earlier retention times when compared with the unoxidised protein. This behavior is consistent with reversed-phase HPLC data on oxidised human growth hormone8, relaxin17-19 and insulin25.
Comparison of the reactivity’s of hydrogen peroxide and peracetic acid show that peracetic acid is approximately 1000 times more reactive than hydrogen peroxide. This is consistent with the enhanced reactivity of a five-centre cyclic transition state that is attainable with the protonated peracetic acid, but is not possible with the peracetic anion or hydrogen peroxide. This is consistent with Chen et al21 which demonstrated that at pH 7, the sulfoxide is preferred, while at basic pH, the sulfone is preferred. Peptide mapping data, not shown, demonstrated that only methionine residues were modified and that only methionine sulfoxide was generated. In addition, data collected on recombinant factor IX (not presented) show that cysteine and cystine residues are not affected by peracetic acid oxidation. Further examination of the peracetic acid oxidation reaction demonstrated that the level of peracetic acid induced oxidation is limited by the oxidant concentration and the oxidation reaction appears to be stoichiometric. These are important considerations in the chemical oxidation of proteins in that it allows for more precise control of the oxidation reaction, allowing for only a certain level of oxidation to occur. Further, when the desired level of oxidation is obtained, no additional quenching of the oxidant, with either catalase9,12,17,19 or N-acetyl-methionine13, or additional sample manipulation is required.
Conclusions
rhIL-11 has two methionine residues, Met58 and Met122. We have demonstrated by chemical oxidation with peracetic acid and hydrogen peroxide that Met58 is more susceptible to oxidation than Met122. This is consistent with long-term stability data collected on aqueous rhIL-11.
Peracetic acid has been demonstrated to be a useful reagent in the chemical oxidation of rhIL-11. The oxidant appears to react stoichiometrically with methionine residues. Further, the level of oxidation can be controlled by limiting the amount of peracetic acid used in the oxidation reaction.
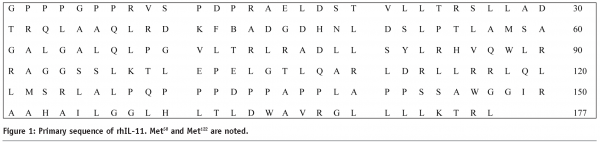
Acknowledgments
The authors would like to acknowledge Marta Czupryn for various discussions and review of this manuscript.
References
- X.X. Du, D.A. Williams: Interleukin-11: a multi functional growth factor derived from the hematopoietic micro environment. Blood, volume 83, issue 8, pp. 2023-2030, 1994.
- M.S. Gordon, etal: Sledge: Recombinant human interleukin eleven (NEUMEGATM rhIL-11 Growth Factor; rhIL-11) prevents severe thrombocytopenia in breast cancer patients receiving multiple cycles of cyclophosphamide (C) and doxorubicin (A) chemotherapy. Proc. ASCO, 13:133, 1994.
- S. Bank, etal: Safety and Activity of rhIL 11 in Subjects With Active Crohn’s Disease. Gastroenterology,112 (4 suppl):A883, 1997.
- S.R. Paul, etal: Molecular cloning of a cDNA encoding interleukin 11, a stromal cell-derived lymphopoietic and hematopoietic cytokine. Proc. Natl. Acad. Sci. USA, 87, pp. 7512-7516, 1990
- T.H. Nguyen: Oxidation degradation of protein pharmaceuticals. In Formulation and Delivery of Proteins and Peptides. Amer Chem. Soc. Washington, DC., pp. 59-71, 1994.
- N. Brot and H. Weissbach: Biochemistry of methionine sulfoxide residues in proteins. BioFactors, volume 3, issue 2, pp. 91-96, 1991.
- K. Rose, P.-O. Regamey, R. Anderegg, T.N.C. Wells and A.E.I. Proudfoot: Human interleukin-5 expressed in Escherichia coli has N-terminal modifications. Biochem. J., 286, pp. 825-828, 1992.
- G. Teshima and E. Canova-Davis: Separation of oxidised human growth hormone variants by reversed-phase high-performance liquid chromatography. Journal of Chromatography 625, pp. 207-215, 1992.
- C.B. Glaser and C.H. Li: Reaction of Bovine Growth Hormone with Hydrogen Peroxide. Biochemistry, volume 13 issue 5, pp. 1044-1047, 1974.
- K. Sasaoki, T. Hiroshima, S. Kusumoto and K. Nishi: Oxidation of methionine residues of recombinant human interleukin 2 in aqueous solutions. Chem. Pharm Bull volume 37, issue 8, pp. 2160-2164, 1989.
- R.A. Houghton, C.B. Glaser and C.H. Li: Human Somatotropin. Archives of Bioch. and Biophys, 178, pp. 350-355, 1977.
- L.C. Teh, etal: Methionine oxidation in human growth hormone and human chorionic somatomammotropin. J. Biol. Chem., 262(14), pp. 6447- 6472, 1987.
- Y. Schechter, Y. Burstein and A. Patchornick: Selective oxidation of methionine residues in proteins. Biochemistry 14(20), pp. 4497-4503, 1975.
- C. Nishimura, etal:Chemical modification and 1H-NMR studies on the receptor-binding region of human interleukin-6. Eur. J. Biochem, 196(2), pp. 377-384, 1991.
- V. Mihajlovic, O. Cascone and M.J. Biscoglio De Jiménex Bonino: Oxidation of methionine residues in equine growth hormone by chloromine-T. Int. J. Biochem, 25(8), pp. 1189-1193, 1993.
- S.-T. Chu, C.-C. Chu, C.-C. Tseng and Y.-H. Chen: Met-8 of the β1-bungarotoxin phospholipase A2 subunit is essential for the phopholipase A2-independent neurotoxic effect. Biochem. J., 295, pp. 713-718, 1993.
- T.E. Nguyen, J. Burnier and W. Meng: The kinetics of relaxin oxidation by hydrogen peroxide. Pharm. Res., 10(11), pp. 1563-1571, 1993.
- D.C. Cipolla and S.J. Shire: Analysis of oxidised human relaxin by reverse phase HPLC, mass spectrometry and bioassays. In J.J. Villafrance Ed. Techniques in Protein Chemistry II, Academic Press, San Diego, pp. 543-555, 1991.
- S. Li, T.H. Nguyen, C. Schöneich and R.T. Borchardt. Aggregation and precipitation of human relaxin induced by metal-catalyzed oxidation. Biochemistry, 34, pp. 5762-5772, 1995.
- R.G. Keck: The use of t-Butyl hydroperoxide as a probe for methionine oxidation in proteins. Anal. Biochem., 236, pp. 56-62, 1996.
- S.H. Chen, R.A. Kenley and J.S. Winterle: Sulconazole reactions with peracetic acid and hydrogen peroxide. Int’l. Jour. of Pharm, Vol 72, pp. 89-96, 1991.
- R.J.W. Lambert, M.D.Johnston and E.A. Simons. A kinetic study of the effect of hydrogen peroxide and peracetic acid against Staphylococcus aureus and Pseudomonas aeruginose using the Bioscreen disinfection method. Journal of Applied Microbiology 1999, Vol 87, pp. 782-786, 1999.
- M. Czupryn, etal: Alanine-scanning Mutagenesis of Human Interleukin-11: Identification of Regions Important for Biological Activity. in Interleukin-6 Type Cytokines, Volume 762 of the Annals of the New York Academy of Sciences, July 21, 1995
- M.J. Czupryn, J.M. McCoy and H.A. Scoble. Structure-Function Relationships in Human Interleukin-11. J. Biol. Chem., 270(2), pp. 978-985, 1995.
- D. Gross, etal: Oxidation of rat insulin II, but not I, leads to anomalous elution profiles upon HPLC analysis of insulin-related peptides. FEBS, 241, pp. 205-208, 1988.