Rapid microbiological methods: A looking glass into what and how we are
Posted: 25 June 2020 | Lucpah Nekati (Graduate microbiologist) | No comments yet
The impact of rapid microbiological methods (RMM) on the scientific community has been widespread and profound. Lucpah Nekati explains how their numerous benefits enable deeper understanding, faster and more easily.
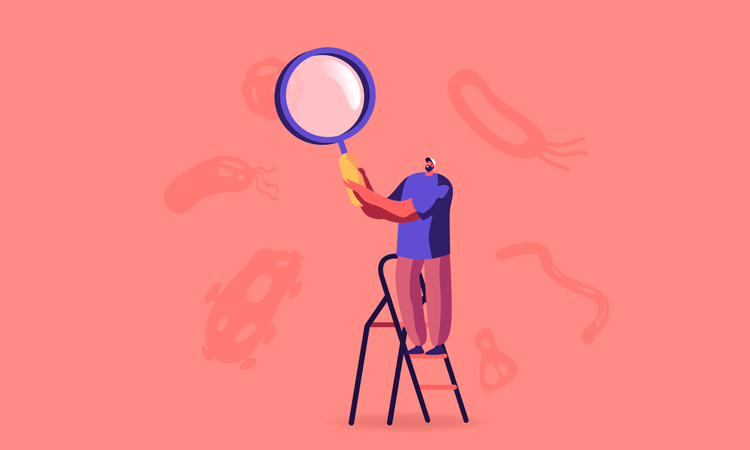
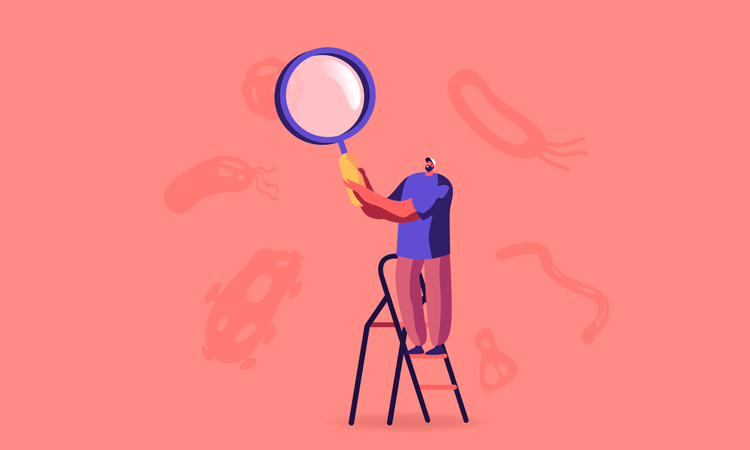
RMM ARE increasingly becoming popular in the pharmaceutical, clinical, agricultural, food and processing sectors. The ease of use, sensitivity, specificity, cost effectiveness and reduced preparation and running time all contribute to its prevalence over traditional or culture-based methods. Nucleic acid assays have shown to be the favourable method, as their mechanism allows detection of other genes and biomarkers aside from the targeted pathogens. Metagenomics in diagnosis has been commended for minimal bias methods of detecting pathogens as direct sequencing of sample DNA is involved. This article will briefly discuss the implementation of molecular RMM in diagnosis and in studying microbiomes.
With metagenomics, it has become possible to analyse samples without the tedious procedures of culturing microbes and extracting DNA. Instead, DNA is obtained directly from samples with subsequent amplification and sequencing processes.1 This provides a shorter, easier, specific and sensitive route for analysing sample DNA. Rapid scientific response, such as in the 2011 Europe E. coli outbreak, has become possible with the advancement of genomic studies such as whole-genome sequencing.3 Targeted sequencing like 16S rRNA-gene sequencing is becoming popular in disease diagnosis as these methods can assist in identifying complex infections and in tracing the origins of an infection, which may pave the way for appropriate treatment regimens for patients. This is especially true with gut microbes, most of which are unculturable; and consequently 16S rRNA-gene sequencing is gaining popularity in clinical microbiology labs.4 Aside from the detection of pathogenic microbes, 16S rRNA-gene sequencing may also enable the identification of other microorganisms that potentially affect the gut health of patients.5 Furthermore, there are other factors at play, such as virulence and interpretation of functional pathways for expounding multifaceted illnesses.1 A single assay of RMM such as FilmArray can allow the detection of as many as 22 microbes.1 The 16S rRNA-gene sequencing molecular technique has proven to be one of the most essential developments of the 21st century. Sequencing of the 16S rRNA gene allows studies in diverse metagenomic samples.6 This has created further opportunities, providing for close investigation of microbiomes.
The ease of use, sensitivity, specificity, cost effectiveness and reduced preparation and running time all contribute to its prevalence over traditional or culture-based methods”
The 16S rRNA gene is conserved in most bacteria and is the target gene in most molecular-based DNA metagenomics.7,8 The advent of molecular-based DNA metagenomics has enabled the identification of several bacteria belonging to the gut microbiome – most of which belong to the unculturable group.9 Better insights into the microbiome and microbiome-host relationships have been gained with the help of advancements in DNA molecular techniques that are continuously being used to study microbiomes.10
Prior to the introduction and increased adoption of RMM, slow culture-dependent methods that could not identify most microbes due to their limited cultivability were used.11 With shotgun metagenomics as a diagnostic tool, it is now possible to identify previously uncharacterised microbes and/or emergent and novel pathogens.12 Furthermore, these molecular-based DNA techniques have gone the extra mile by identifying antimicrobial resistance genes within microbiomes.13 Some of these techniques, like Resistome Capture, ResCap, can assist with identifying antimicrobial genes with very low specificity and sensitivity.13
In addition to being important reservoirs of antimicrobial resistance genes, the microbiome, according to various studies, has been shown as essential to human health. Some recent studies have demonstrated the association of the gut microbiome to various mental health issues such as depression and anxiety.14 Other lifestyle choices like alcohol dependence have been attributed to influence from the gut microbiome. These findings would still be speculations and concerns of the future were it not for next‑generation sequencing that has been the result of development of RMM.
Conclusion
The introduction and adoption of RMM, or rather culture-independent diagnosis tests, has revolutionised the science industry. Not only is faster, more reliable and cost-effective diagnosis now possible, but the methods also enable the detection of complex infections and antimicrobial resistance. Next-generation sequencing has marked a milestone and triggered more studies of microbiomes.
About the author
Lucpah Nekati gained a Master’s degree in medical microbiology and virology from the University of KwaZulu-Natal. Her research interests include antimicrobial resistance, bioinformatics and microbiome analysis.
References
- FORBES, J. D., KNOX, N. C., RONHOLM, J., PAGOTTO, F. & REIMER, A. 2017. Metagenomics:
the next culture-independent game changer. Frontiers in microbiology, 8, 1069. - OAKLEY, B. B., LILLEHOJ, H. S., KOGUT, M. H., KIM, W. K., MAURER, J. J., PEDROSO, A., LEE, M.
D., COLLETT, S. R., JOHNSON, T. J. & COX, N. A. 2014. The chicken gastrointestinal microbiome. FEMS
microbiology letters, 360, 100-112. - GRAD, Y. H., LIPSITCH, M., FELDGARDEN, M., ARACHCHI, H. M., CERQUEIRA, G. C., FITZGERALD, M., GODFREY, P., HAAS, B. J., MURPHY, C. I. & RUSS, C. 2012. Genomic epidemiology of the Escherichia coli O104: H4 outbreaks in Europe, 2011. Proceedings of the National Academy of Sciences, 109, 3065-3070.
- WEINSTOCK, G. M. 2012. Genomic approaches to studying the human microbiota. Nature, 489, 250.
- ALMONACID, D. E., KRAAL, L., OSSANDON, F. J., BUDOVSKAYA, Y. V., CARDENAS, J. P., BIK, E. M.,
GODDARD, A. D., RICHMAN, J. & APTE, Z. S. 2017. 16S rRNA gene sequencing and healthy reference
ranges for 28 clinically relevant microbial taxa from the human gut microbiome. PloS one, 12, e0176555. - RAM, J. L., KARIM, A. S., SENDLER, E. D. & KATO, I. 2011. Strategy for microbiome analysis using
16S rRNA gene sequence analysis on the Illumina sequencing platform. Systems biology in reproductive medicine, 57, 162-170. - GRICE, E. A. 2015. The intersection of microbiome and host at the skin interface: genomic-and
metagenomic-based insights. Genome research, 25, 1514-1520. - MACCAFERRI, S., BIAGI, E. & BRIGIDI, P. 2011. Metagenomics: key to human gut microbiota.
Digestive diseases, 29, 525-530. - TURNBAUGH, P. J., HAMADY, M., YATSUNENKO, T., CANTAREL, B. L., DUNCAN, A., LEY, R.
E., SOGIN, M. L., JONES, W. J., ROE, B. A. & AFFOURTIT, J. P. 2009. A core gut microbiome in
obese and lean twins. nature, 457, 480. - WEESE, J. S., SLIFIERZ, M., JALALI, M. & FRIENDSHIP, R. 2014. Evaluation of the nasal microbiota in slaughter-age pigs and the impact on nasal methicillin-resistant Staphylococcus aureus (MRSA) carriage. BMC veterinary research, 10, 69.
- ZEINELDIN, M., ALDRIDGE, B. & LOWE, J. 2018. Dysbiosis of the fecal microbiota in feedlot cattle with hemorrhagic diarrhea. Microbial pathogenesis, 115, 123-130.
- BROWNE, H. P., FORSTER, S. C., ANONYE, B. O., KUMAR, N., NEVILLE, B. A., STARES, M. D.,
GOULDING, D. & LAWLEY, T. D. 2016. Culturing of ‘unculturable’human microbiota reveals novel taxa
and extensive sporulation. Nature, 533, 543. - LANZA, V. F., BAQUERO, F., MARTÍNEZ, J. L., RAMOS-RUIZ, R., GONZALEZ-ZORN, B., ANDREMONT, A., SANCHEZ-VALENZUELA, A., EHRLICH, S. D., KENNEDY, S. & RUPPE, E. 2018.
In-depth resistome analysis by targeted metagenomics. Microbiome, 6, 11. - LECLERCQ, S., STÄRKEL, P., DELZENNE, N. M. & DE TIMARY, P. 2018. The gut microbiota: a new target in the management of alcohol dependence? Alcohol.
- JACKSON, B. R., TARR, C., STRAIN, E., JACKSON, K. A., CONRAD, A., CARLETON, H., KATZ, L. S.,
STROIKA, S., GOULD, L. H. & MODY, R. K. 2016. Implementation of nationwide real-time wholegenome sequencing to enhance listeriosis outbreak detection and investigation. Reviews of Infectious Diseases, 63, 380-386.
Issue
Related topics
Analytical techniques, metagenomics, Microbiology, QA/QC, Rapid Microbiological Methods (RMMs), RNA