How can NMR spectroscopy help protein biopharmaceutical development?
Posted: 24 February 2020 | Dr Alexander Golovanov (University of Manchester), Jack Bramham (University of Manchester) | No comments yet
Nuclear magnetic resonance (NMR) spectroscopy is a technique that most have heard of but many avoid, especially when dealing with larger biological macromolecules. Why? The common wisdom is that NMR can be complicated, expensive and involves quantum physics and complex equations. In the fast-paced industrial biopharmaceutical environment there may be a disinclination to expend the necessary time and resources to set up the technique, but Jack Bramham and Alexander Golovanov highlight here some reasons why it may be worth the investment.
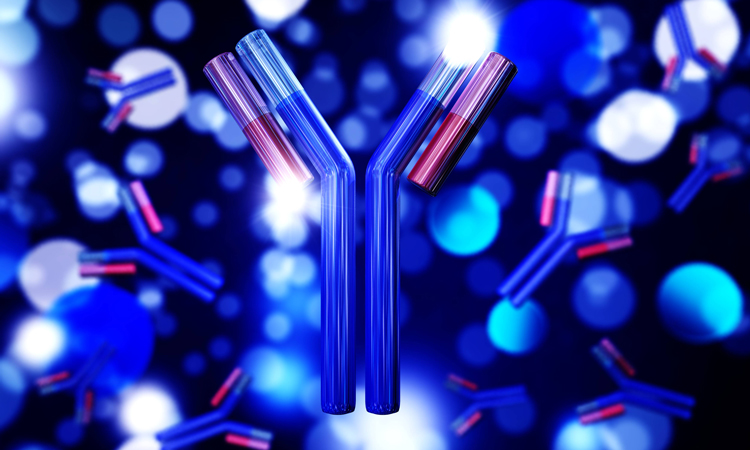
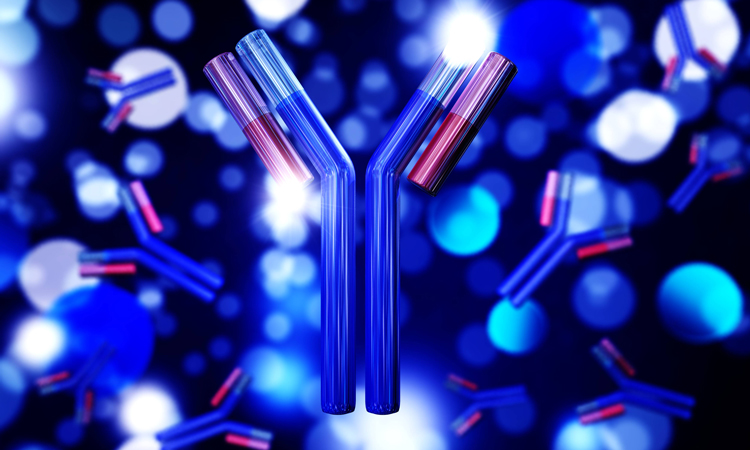
THE SUBJECT of NMR may bring to mind Schrödinger equations, Hamiltonians and spin states. For non-specialists, these may appear too distant from the more prosaic day-to-day tasks of a bioprocessing or formulation scientist, such as how to make a biopharmaceutical formulation better, more stable, with increased shelf life and decreased aggregation. Since their introduction in 1982, protein-based biopharmaceuticals have become increasingly important therapies in the treatment of a wide range of diseases, including cancer, autoimmune and blood clotting diseases. Recent years have seen the advancement of more complex monoclonal antibody (mAb) products and the emergence of new protein-based engineered modalities, often applied as high concentration formulations or as co-formulations of several proteins together.
Antibody-drug conjugates, mAbs and new modalities of ever increasing complexity may suffer from issues typical for any protein; that is, under certain conditions (eg, temperature, pH or over time) they may become destabilised and, due to inherent general ‘stickiness’ of their surface, proteins may form reversible assemblies or clusters, causing an increase in formulation viscosity, or form irreversible aggregates. In addition, protein solutions may undergo liquid-liquid phase separation, which can initially manifest as sample opalescence due to suspended dense liquid droplets, and later as formation of distinct protein-rich and lean layers in the sample. With all these problems to overcome, the quantum physics of NMR may not appear so scary after all. The complexity of biopharmaceutical entities and their formulations, and the multitude of possible things that can go wrong at the initial research stages, requires new approaches and orthogonal techniques that can deal with this complexity in situ in biopharmaceutical formulation. This is where the power of NMR comes into play.
While NMR is routinely used in small molecule pharmaceutical development for assessment of chemical structure, purity and stability, it is less widely used in biopharmaceutical development. However, as we demonstrate here, NMR is a powerful technique, which by itself or in combination with orthogonal techniques, can be used to address a wide variety of biopharmaceutical issues.
Protein-based biopharmaceuticals have become increasingly important therapies in the treatment of a wide range of diseases”
The isotopes 1H, 13C, 15N and 19F are NMR-visible and are the most relevant for biopharmaceutical molecules. The most abundant, 1H, is present in all proteins as well as in most excipients, leading to significant signal overlap in one-dimensional 1H spectra. While these spectra may be complex for large proteins like mAbs, useful information can still be obtained.1 Particular regions of mAbs spectra free from excipient signals can be used to study protein behaviour via various NMR-measured parameters. In NMR, signal intensity reports on the concentration of atoms from which the signal is observed. For example, signal intensity can be used to determine protein concentration once the protein is denatured.2 In non-denatured protein solutions, signal intensity is affected by a number of factors, including viscosity and self-association. Therefore, by accounting for viscosity and protein concentration, 1H NMR signal intensity can report on mAb self-association, for example, in the presence of different excipients.1 The relaxation and translational diffusion measured for 1H signal of the mAb can also be independently used to characterise protein behaviour in solution, such as self-association.3-4 Alternatively, if the signals from small molecule excipients present in the same sample are of interest, spectral complexity can be reduced by using ‘relaxation filters’ to remove fast-relaxing signals from protein components, leaving only the slower relaxing small molecule signals. These filtered spectra can be used to detect extractables and leachables from bioprocessing5 or track the success of dialysis.6
Incorporating 13C and/or 15N labels in proteins, often in combination with multi-dimensional NMR spectroscopy, is a method routinely used to address complex questions about protein structure and dynamics. Proteins expressed in E. coli or common yeast systems can be easily enriched with these isotopes, permitting such experiments. While for many mAbs produced in mammalian cells this may not be a feasible strategy, advances in NMR hardware and techniques have enabled experiments to be conducted on these isotopes present at natural abundance. Perhaps the most prominent recent application of NMR to biopharmaceuticals is the ‘fingerprinting’ of protein higher order structure (HOS) in mAbs performed without any isotopic labelling.7-8 Like human fingerprints, these NMR fingerprints are unique to an individual mAb sequence and HOS, so can be used to directly compare protein identities. This approach is particularly relevant for biosimilars – generic versions of mAbs, which are on the rise following expiration of early patents. Although biosimilars may have an identical primary sequence to their reference mAbs, it is important for efficacy and safety to ensure that post-translational modifications and protein folding result in similar mAb HOS. NMR fingerprinting allows biopharmaceutical companies and regulators to confirm this, as well as to check batch‑to-batch variabilities.
The various types of NMR instruments available make it a versatile technique for addressing a broad range of formulation problems. Solid‑state NMR can be used to study stabilisation by excipients in solid state formulations16 and to quantify residual moisture after freeze drying.17 Alternatively, imaging instruments – similar to magnetic resonance imaging (MRI) scanners found in hospitals – can be used to study dynamic processes that occur differently across a sample, such as the reconstitution of a solid mAb formulation for injection.18 Finally, benchtop NMR spectrometers can be used to indirectly detect a number of biopharmaceutical protein properties, such as protein concentration or aggregation, based on the behaviour of the solvent water NMR signal.19-20 These much smaller instruments can be incorporated into conventional formulation labs or production lines for inline monitoring using ‘flow’ NMR to detect the emergence of changes, alerting on potential problems in the process.21
The various types of NMR instruments available make NMR a versatile technique for addressing a broad range of formulation problems”
Recently, the use of 19F NMR has become increasingly popular; although these atoms are not native parts of protein molecules, they can be incorporated either using non-natural amino acids or by sparsely attaching 19F-containing tags to the protein surface via chemical linkage to cysteine or lysine sidechains. One advantage of observing 19F NMR signals from such probes, which represent the behaviour of the whole molecule, is that there are no other signals in the background. We have recently demonstrated the use of 19F tags to characterise the behaviour of individual mAbs or proteins in complex mixtures22 or co-formulations.23 By differentially labelling each mAb with a specific 19F tag with a unique characteristic chemical shift, the behaviour of the observed signal can be unambiguously linked with the behaviour of a specific protein molecule and tracked under a range of conditions. This technique makes it possible to characterise mAb-specific clustering in co-formulations24 and to optimise formulations to prevent such clustering. Untangling such complexity present in biopharmaceutical formulation samples in situ – from the viewpoint of different components, both large and small – is what NMR does best and is a capability that may be difficult to achieve by any other single biophysical technique.
About the authors
References
- Kheddo P, Cliff MJ, Uddin S, van der Walle CF, Golovanov AP. Characterizing monoclonal antibody formulations in arginine glutamate solutions using 1H NMR spectroscopy. mAbs 2016, 8 (7), 1245-1258.
- Bradley SA, Jackson WC, Mahoney PP. Measuring Protein Concentration by Diffusion-Filtered Quantitative Nuclear Magnetic Resonance Spectroscopy. Analytical Chemistry 2019, 91 (3), 1962-1967.
- Kheddo P, Bramham JE, Dearman RJ, Uddin S, van der Walle CF, Golovanov AP. Investigating Liquid-Liquid Phase Separation of a Monoclonal Antibody Using Solution-State NMR Spectroscopy: Effect of Arg center dot Glu and Arg center dot HCI. Mol. Pharm. 2017, 14 (8), 2852-2860.
- Falk BT, Liang YK, Bailly M, Raoufi F, Kekec A, Pissarnitski D, Feng D, Yan L, Lin SN, Fayadat-Dilman L, McCoy MA. NMR Assessment of Therapeutic Peptides and Proteins: Correlations That Reveal Interactions and Motions. Chembiochem 2019, 6.
- Skidmore K, Hewitt D, Kao Y-H. Quantitation and characterization of process impurities and extractables in protein-containing solutions using proton NMR as a general tool. Biotechnol. Prog. 2012, 28 (6), 1526-1533.
- Magarian N, Lee K, Nagpal K, Skidmore K, Mahajan E. Clearance of Extractables and Leachables from Single-Use Technologies via Ultrafiltration/Diafiltration Operations. Biotechnol. Prog. 2016, 32 (3), 718-724.
- Arbogast LW, Brinson RG, Marino JP. Application of Natural Isotopic Abundance 1H–13C- and 1H–15N-Correlated Two-Dimensional NMR for Evaluation of the Structure of Protein Therapeutics. In Methods in Enzymology, Academic Press: 2015.
- Brinson RG, et al. Enabling adoption of 2D-NMR for the higher order structure assessment of monoclonal antibody therapeutics. Mabs 2019, 11 (1), 94-105.
- Hinterholzer A, Stanojlovic V, Cabrele C, Schubert M. Unambiguous Identification of Pyroglutamate in Full-Length Biopharmaceutical Monoclonal Antibodies by NMR Spectroscopy. Analytical Chemistry 2019, 91 (22), 14299-14305.
- Bandi S, Singh SM, Shah DD, Upadhyay V, Mallela KMG. 2D NMR Analysis of the Effect of Asparagine Deamidation Versus Methionine Oxidation on the Structure, Stability, Aggregation, and Function of a Therapeutic Protein. Mol. Pharm. 2019, 16 (11), 4621-4635.
- Majumder S, Saati A, Philip S, Liu LL, Stephens E, Rouse JC, Ignatius AA. Utility of High Resolution NMR Methods to Probe the Impact of Chemical Modifications on Higher Order Structure of Monoclonal Antibodies in Relation to Antigen Binding. Pharm Res 2019, 36 (9), 13.
- Peng JN, Patil SM, Keire DA, Chen K. Chemical Structure and Composition of Major Glycans Covalently Linked to Therapeutic Monoclonal Antibodies by Middle-Down Nuclear Magnetic Resonance. Analytical Chemistry 2018, 90 (18), 11016-11024.
- Singh SM, Bandi S, Jones DNM, Mallela KMG. Effect of Polysorbate 20 and Polysorbate 80 on the Higher-Order Structure of a Monoclonal Antibody and Its Fab and Fc Fragments Probed Using 2D Nuclear Magnetic Resonance Spectroscopy. Journal of Pharmaceutical Sciences 2017, 106 (12), 3486-3498.
- Morales MM, Zalar M, Sonzini S, Golovanov AP, van der Walle CF, Derrick JP. Interaction of a Macrocycle with an Aggregation-Prone Region of a Monoclonal Antibody. Mol. Pharm. 2019, 16 (7), 3100-3108.
- Svilenov HL, Kulakova A, Zalar M, Golovanov AP, Harris P, Winter G. Orthogonal Techniques to Study the Effect of pH, Sucrose, and Arginine Salts on Monoclonal Antibody Physical Stability and Aggregation During Long-Term Storage. Journal of Pharmaceutical Sciences 2020, 109 (1), 584-594.
- Mensink MA, Nethercott MJ, Hinrichs WLJ, van der Voort Maarschalk K, Frijlink HW, Munson EJ, Pikal MJ. Influence of Miscibility of Protein-Sugar Lyophilizates on Their Storage Stability. Aaps j 2016, 18 (5), 1225-1232.
- Abraham A, Elkassabany O, Krause ME, Ott A. A nondestructive and noninvasive method to determine water content in lyophilized proteins using low-field time-domain NMR. Magnetic Resonance in Chemistry 2019, 57 (10), 873-877.
- Partridge TA, Ahmed M, Choudhary SB, van der Walle CF, Patel SM, Bishop SM, Mantle MD. Application of Magnetic Resonance to Assess Lyophilized Drug Product Reconstitution. Pharm Res 2019, 36 (5), 71.
- Taraban MB, Briggs KT, Yu YB. Magnetic Resonance Relaxometry for Determination of Protein Concentration and Aggregation. Current protocols in protein science 2020, 99 (1), e102.
- Taraban MB, DePaz RA, Lobo B, Yu YB. Water Proton NMR: A Tool for Protein Aggregation Characterization. Analytical Chemistry 2017, 89 (10), 5494-5502.
- Taraban MB, Briggs KT, Merkel P, Yu YB. Flow Water Proton NMR: In-Line Process Analytical Technology for Continuous Biomanufacturing. Analytical Chemistry 2019, 91 (21), 13538-13546.
- Edwards JM, Harris P, Bukrinski JT, Golovanov AP. Use of 19F Differential Labelling for the Simultaneous Detection and Monitoring of Three Individual Proteins in a Serum Environment. ChemPlusChem 2019, 84 (5), 443-446.
- Edwards JM, Derrick JP, van der Walle CF, Golovanov AP. 19F NMR as a Tool for Monitoring Individual Differentially Labeled Proteins in Complex Mixtures. Mol. Pharm. 2018, 15 (7), 2785-2796.
- Edwards JM, Bramham JE, Podmore A, Bishop SM, van der Walle CF, Golovanov AP. 19F Dark-State Exchange Saturation Transfer NMR Reveals Reversible Formation of Protein-Specific Large Clusters in High-Concentration Protein Mixtures. Analytical Chemistry 2019, 91 (7), 4702-4708.