Polyelectrolyte multilayering: an emerging class of carriers for therapeutic agents
Posted: 20 February 2020 | Paul Royall (King’s College London), Sumayah Abdul-Jabbar (King's College London) | No comments yet
Polyelectrolyte multilayers (PEMs) are promising drug carriers with potential applications in the delivery of poorly soluble drugs. In this article, Sumayah Abdul-Jabbar and Paul Royall discuss the approaches for PEM construction and drug release and detail several applications of multilayered particles in therapeutics.
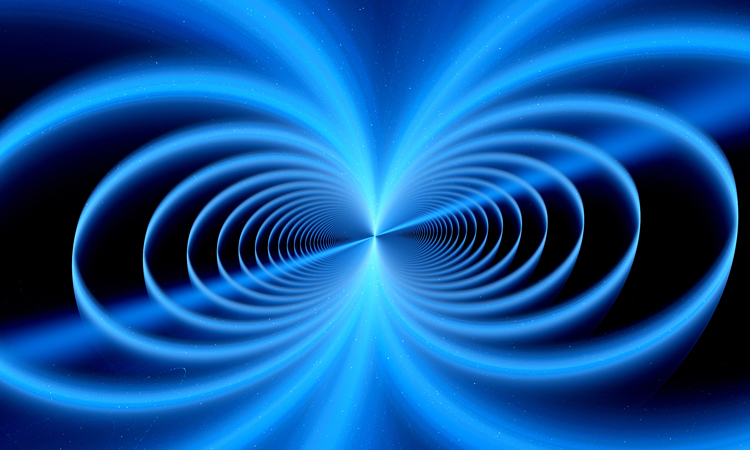
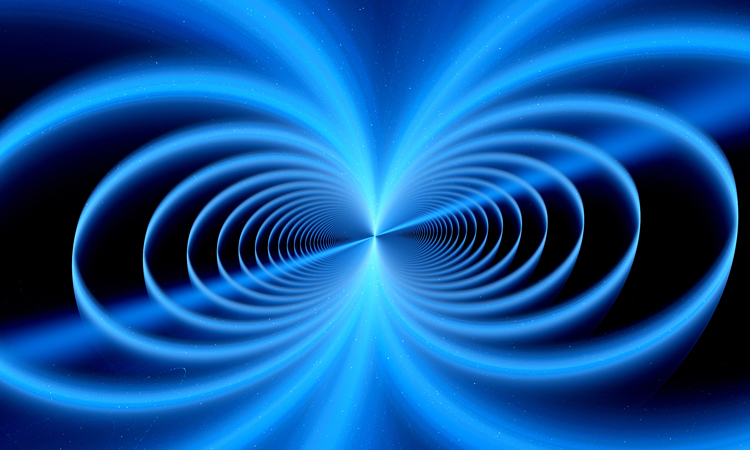
PEMs are novel vesicles that have proved to be promising drug delivery vehicles for drug encapsulation”
A POPULAR APPROACH to solubilise poorly soluble drugs is encapsulating them within polymers by forming micelles or liposomes.1 However, there are limitations associated with these carriers, such as low-loading efficiency of the drug, difficulties with scaling up and inadequate storage stability. PEMs are novel vesicles that have proved to be promising drug delivery vehicles for drug encapsulation.3 PEMs are designed by the stepwise deposition of polymer layers; therefore, particles can be tailored during fabrication to vary properties. This enables the formulation of versatile materials with multiple functionalities and a variety of properties.4 In addition to drug delivery, they are used in bio-sensing, regenerative medicine, tissue engineering, bio-mimetics research5 and diagnostic and theranostic applications.6 The European patent office holds patent applications for the use of PEMs in biosensors (priority number: KR20090041461 20090513) as well as in controlled and sustained release (priority number: EP20000118615 20000828).
Polyelectrolytes themselves play an important role in drug delivery as, in addition to the formation of multilayers, they are also recognised for the formation of complexes for drug delivery.7 PEMs contribute towards both steric and electrostatic interactions, which collectively enhance the physical and chemical stability of colloids when compared to those prepared by other approaches.8 The construction of a PEM is mainly driven by electrostatic interactions. These layered structures are a consequence of the alternating exposure of a charged substrate to solutions of positive or negative polyelectrolytes, whereby each adsorption step leads to a charge inversion of the surface (Figure 1) – the approach is often referred to as layer-by-layer.9
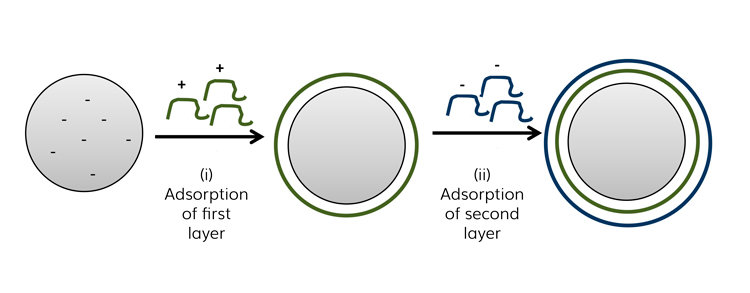
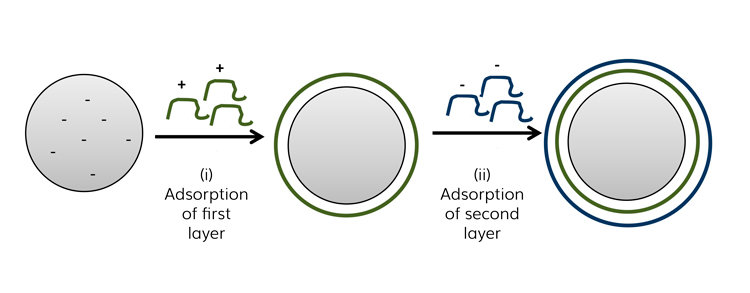
Figure 1: Schematic representation of the layer-by-layer. The first step (i) shows adsorption of a positively charged polyelectrolyte onto an oppositely negatively charged colloidal template. In the second step (ii), a subsequent polyelectrolyte layer with a negative charge, opposite to the first one, was adsorbed. This process is repeated until the desired number of layers is achieved.
The approaches for PEM construction are versatile and can be broadly categorised into five distinct routes of assembly: immersive, spin, spray, fluidic and electromagnetic.
Spin assembly utilises the common coating technology of spinning a substrate to facilitate the deposition of materials.10 Spray assembly constructs layers by aerosolising polymer solutions and sequentially spraying them onto substrates.11 Fluidic assembly involves using pressure or vacuum to build multilayers using fluidics that are pushed through a capillary12 utilising specialised equipment. Electromagnetic assembly is based on the use of an applied electric or magnetic field to perform layering.13
Deposition of PEMs onto colloidal substrates is typically monitored by electrophoresis by determining zeta potential after each layer, whereby zeta potential oscillates between positive and negative values after each layer deposition.16 Deposition can also be monitored by evaluating the layer thickness9 using ellipsometry,17 flow cytometry,14 atomic force microscopy and surface plasmon resonance spectroscopy.18 The morphology can be examined using atomic force microscopy, scanning electron microscopy and transmission electron microscopy, while the film stiffness and permeability can be determined using confocal laser scanning microscopy and atomic force microscopy.14
It is apparent from the formulation method that the particles can be tailored during fabrication to achieve various properties such as size, composition, porosity, surface functionality and colloidal stability resulting in a tuneable approach for generating functional films for a variety of applications.
There are two main approaches for drug release (Figure 2). Furthermore, tailoring the number of layers and type of polymers used can achieve both controlled and sustained drug delivery.19
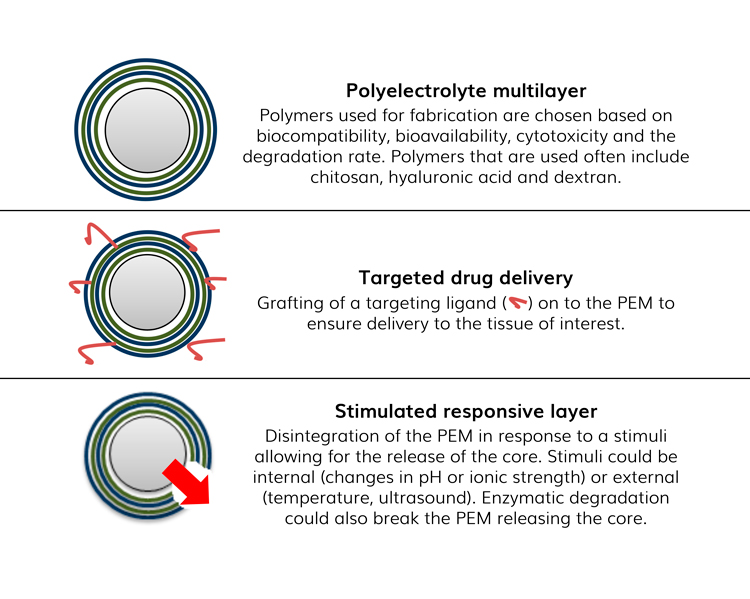
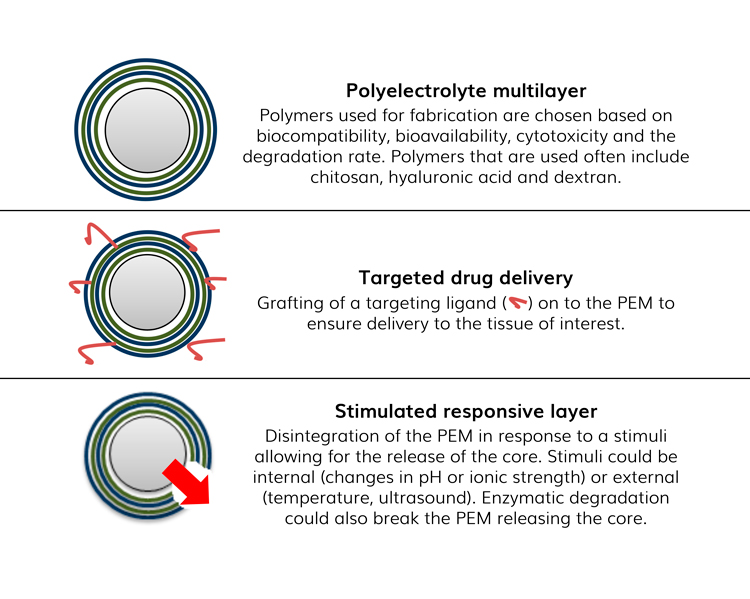
Figure 2: Approaches for drug release from PEMs
There are numerous applications for PEMs: multilayered artemisinin showed improved hydrophilicity and stability;3 multilayered paclitaxel nanoparticles resulted in stable nanocolloids with very high drug loadings, controlled drug release rates and the possibility of attaching targeting ligands;2 and silica particles were coated with alternating layers of polymers linked to either doxorubicin or paclitaxel providing biodegradable microcapsules with a greater specificity to cancer cells. Furthermore, these drug systems showed an efficient cellular uptake by multidrug resistant colorectal cancer cells in vitro.20
Gold nanoparticles were coated with the oppositely charged polyelectrolytes siRNA and poly (ethyleneimine). This process offered a unique opportunity to fabricate well-defined and homogenously distributed nanocarriers for siRNA delivery.26 Layer-by-layer assembled nanoengineered hydrogel capsules represented a novel and promising technology for the protection and delivery of labile vaccine candidates to antigen‑presenting cells. Proteins, as well as peptides, were successfully encapsulated within nanoengineered capsules.27 Most recently, polyelectrolyte multilayers have demonstrated a role in antimicrobial therapy.28-30
In conclusion, layer-by-layer assembly is a simple process for fabrication leading to one of the most widely accepted platforms for incorporating molecules with nanometre precision.31 In addition, the application of multilayered particles in therapeutics is rapidly emerging, providing an avenue for obtaining multifunctional, biocompatible and biodegradable coatings that are valuable in drug delivery.
About the authors
References
- Torchilin VP. Micellar nanocarriers: Pharmaceutical perspectives. Pharm Res. 2007 JAN 2007;24(1):1-16.
- Agarwal A, Lvov Y, Sawant R, Torchilin V. Stable nanocolloids of poorly soluble drugs with high drug content prepared using the combination of sonication and layer-by-layer technology. J Controlled Release. 2008 JUN 24 2008;128(3):255-60.
- Chen Y, Lin X, Park H, Greever R. Study of artemisinin nanocapsules as anticancer drug delivery systems. Nanomedicine-Nanotechnology Biology and Medicine. 2009 SEP 2009;5(3):316-22.
- Johnston APR, Cortez C, Angelatos AS, Caruso F. Layer-by-layer engineered capsules and their applications. Current Opinion in Colloid & Interface Science. 2006 OCT 2006;11(4):203-9.
- Poon Z, Lee JB, Morton SW, Hammond PT. Controlling in Vivo Stability and Biodistribution in Electrostatically Assembled Nanoparticles for Systemic Delivery. Nano Letters. 2011 MAY 2011;11(5):2096-103.
- Correa S, Dreaden EC, Gu L, Hammond PT. Engineering nanolayered particles for modular drug delivery. J Controlled Release. 2016 OCT 28;240:364-86.
- Anita Umerska. Polyelectrolyte complexes as nanoparticulate drug delivery systems. European pharmaceutical review. 2015.
- Caruso F. Generation of complex colloids by polyelectrolyte-assisted electrostatic self-assembly. Aust J Chem. 2001 2001;54(6):349-53.
- Schonhoff M. Self-assembled polyelectrolyte multilayers. Current Opinion in Colloid & Interface Science. 2003 MAR 2003;8(1):86-95.
- Thomas IM. Single-Layer Tio2 and Multilayer Tio2-Sio2 Optical Coatings Prepared from Colloidal Suspensions. Appl Opt. 1987 NOV 1;26(21):4688-91.
- Schlenoff J, Dubas S, Farhat T. Sprayed polyelectrolyte multilayers. Langmuir. 2000 DEC 26;16(26):9968-9.
- Madaboosi N, Uhlig K, Jaeger MS, Moehwald H, Duschl C, Volodkin DV. Microfluidics as A Tool to Understand the Build-Up Mechanism of Exponential-Like Growing Films. Macromolecular Rapid Communications. 2012 OCT 26;33(20):1775-9.
- Hong X, Li J, Wang M, Xu J, Guo W, Li J, et al. Fabrication of magnetic luminescent nanocomposites by a layer-by-layer self-assembly approach. Chemistry of Materials. 2004 OCT 19;16(21):4022-7.
- Richardson JJ, Cui J, Bjornmalm M, Braunger JA, Ejima H, Caruso F. Innovation in Layer-by-Layer Assembly. Chem Rev. 2016 DEC 14;116(23):14828-67.
- Guzey D, McClements DJ. Formation, stability and properties of multilayer emulsions for application in the food industry. Advances in Colloid & Interface Science. 2006 Dec 21;128:227-48.
- Schwarz B, Schonhoff M. Surface potential driven swelling of polyelectrolyte multilayers. Langmuir. 2002 APR 16;18(8):2964-6.
- Porcel C, Lavalle P, Decher G, Senger B, Voegel J-, Schaaf P. Influence of the polyelectrolyte molecular weight on exponentially growing multilayer films in the linear regime. Langmuir. 2007 FEB 13 2007;23(4):1898-904.
- Kujawa P, Moraille P, Sanchez J, Badia A, Winnik FM. Effect of molecular weight on the exponential growth and morphology of hyaluronan/chitosan multilayers: A surface plasmon resonance spectroscopy and atomic force microscopy investigation. J Am Chem Soc. 2005 JUN 29 2005;127(25):9224-34.
- Saha S, Loo SCJ. Recent developments in multilayered polymeric particles – from fabrication techniques to therapeutic formulations. Journal of Materials Chemistry B. 2015;3(17):3406-19.
- Yan Y, Ochs CJ, Such GK, Heath JK, Nice EC, Caruso F. Bypassing Multidrug Resistance in Cancer Cells with Biodegradable Polymer Capsules. Adv Mater. 2010 DEC 14;22(47):5398.
- Zhou J, Romero G, Rojas E, Ma L, Moya S, Gao C. Layer by layer chitosan/alginate coatings on poly(lactide-co-glycolide) nanoparticles for antifouling protection and Folic acid binding to achieve selective cell targeting. J Colloid Interface Sci. 2010 MAY 15 2010;345(2):241-7.
- Poon Z, Chang D, Zhao X, Hammond PT. Layer-by-Layer Nanoparticles with a pH-Sheddable Layer for in Vivo Targeting of Tumor Hypoxia. Acs Nano. 2011 JUN 2011;5(6):4284-92.
- Cortez C, Tomaskovic-Crook E, Johnston APR, Radt B, Cody SH, Scott AM, et al. Targeting and uptake of multilayered particles to colorectal cancer cells. Adv Mater. 2006 AUG 4 2006;18(15):1998-2003.
- Yoo CY, Seong JS, Park SN. Preparation of novel capsosome with liposomal core by layer-by-Layer self-assembly of sodium hyaluronate and chitosan. Colloids and Surfaces B-Biointerfaces. 2016 AUG 1;144:99-107.
- Shi Q, Qian Z, Liu D, Liu H. Surface Modification of Dental Titanium Implant by Layer-by-Layer Electrostatic Self-Assembly. Frontiers in Physiology. 2017 AUG 7;8:574.
- Elbakry A, Zaky A, Liebkl R, Rachel R, Goepferich A, Breunig M. Layer-by-Layer Assembled Gold Nanoparticles for siRNA Delivery. Nano Letters. 2009 MAY;9(5):2059-64.
- Sexton A, Whitney PG, Chong S, Zelikin AN, Johnston APR, De Rose R, et al. A Protective Vaccine Delivery System for In Vivo T Cell Stimulation Using Nanoengineered Polymer Hydrogel Capsules. Acs Nano. 2009 NOV 2009;3(11):3391-400.
- Belbekhouche S, Bousserrhine N, Alphonse V, Carbonnier B. From beta-cyclodextrin polyelectrolyte to layer-by-layer self-assembly microcapsules: From inhibition of bacterial growth to bactericidal effect. Food Hydrocoll. 2019 OCT;95:219-27.
- Dionisio NdA, de Oliveira Farias EA, Marques TA, Quelemes PV, de Araujo AR, Fonseca FM, et al. Layer-by-layer films based on polyaniline, titanate nanotubes, and cetyl trimethyl ammonium bromide for antifungal coatings. Journal of Coatings Technology and Research. 2019 SEP;16(5):1253-62.
- Kruk T, Golda-Cepa M, Szczepanowicz K, Szyk-Warszynska L, Brzychczy-Wloch M, Kotarba A, et al. Nanocomposite multifunctional polyelectrolyte thin films with copper nanoparticles as the antimicrobial coatings. Colloid Surf B-Biointerfaces. 2019 SEP 1;181:112-8.
- Pahal S, Gakhar R, Raichur AM, Varma MM. Polyelectrolyte multilayers for bio-applications: recent advancements. Iet Nanobiotechnology. 2017 DEC;11(8):903-8.
Issue
Related topics
Analytical techniques, Drug Delivery Systems, Formulation, Ingredients, Microscopy