Personalised medicine: are we ready for the revolution?
Posted: 19 March 2008 | Claudio Carini, F. M.D., Ph.D., FRCPath, F. VP of TM at MDS Pharma and Candida Fratazzi M.D., Senior Director, Altus Pharma | No comments yet
The impact of biomarker technology and biomarker strategies in pharmaceutical development is still in its infancy; but the impact is already proving significant. Biomarker strategy forms the basis for personalised medicine, the industry/regulatory focus centres on improving the success rate and reducing the high attrition rate often encountered in early phases of clinical research. The depth and breadth of knowledge required to successfully implement biomarkers into drug development is generating company collaborations and inclusion of professionals that have not traditionally been part of drug product development.
The impact of biomarker technology and biomarker strategies in pharmaceutical development is still in its infancy; but the impact is already proving significant. Biomarker strategy forms the basis for personalised medicine, the industry/regulatory focus centres on improving the success rate and reducing the high attrition rate often encountered in early phases of clinical research. The depth and breadth of knowledge required to successfully implement biomarkers into drug development is generating company collaborations and inclusion of professionals that have not traditionally been part of drug product development.
The impact of biomarker technology and biomarker strategies in pharmaceutical development is still in its infancy; but the impact is already proving significant. Biomarker strategy forms the basis for personalised medicine, the industry/regulatory focus centres on improving the success rate and reducing the high attrition rate often encountered in early phases of clinical research. The depth and breadth of knowledge required to successfully implement biomarkers into drug development is generating company collaborations and inclusion of professionals that have not traditionally been part of drug product development.
Today, we are witnessing a revolution in the understanding of health and disease, spurred on by the sequencing of the human genome and the subsequent creation of a map of human genetic variation. This revolution has been given a name: personalised medicine.
What is personalised medicine?
Personalised medicine means creating the right treatment at the right dose, for the right patient, at the right time and for the right outcome. It is essential to remember that even with the best ideas, understanding technology is crucial to getting innovative drugs to the clinic. To develop the necessary tools and biomarkers, industry and academia need to increase collaborative efforts; personalised medicine is a key factor in this process.
The exact definition of personalised medicine is still in a state of flux. It typically refers to the translation of basic research into actual medical treatment and patient care; often called ‘bench to bedside’ medical development. Personalised medicine can also be more broadly defined referring to the development and application of new technologies, where the emphasis is on early patient testing and evaluation. Personalised medicine is a branch of medical research that attempts to more directly connect basic research to patient care. Personalised medicine refers to using information about a person’s genetic make-up to tailor strategies for the detection, treatment or prevention of disease (Figures 2 and 3).
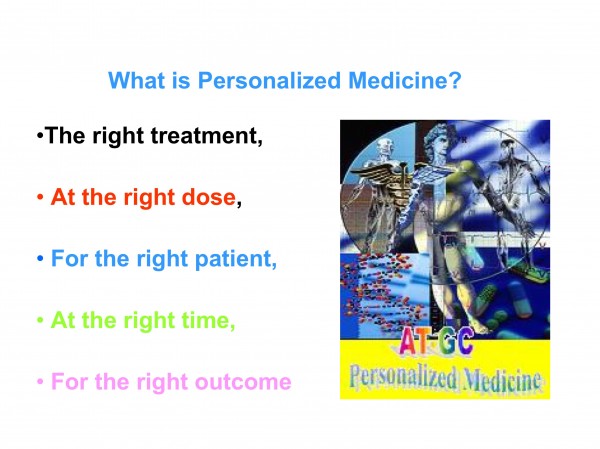
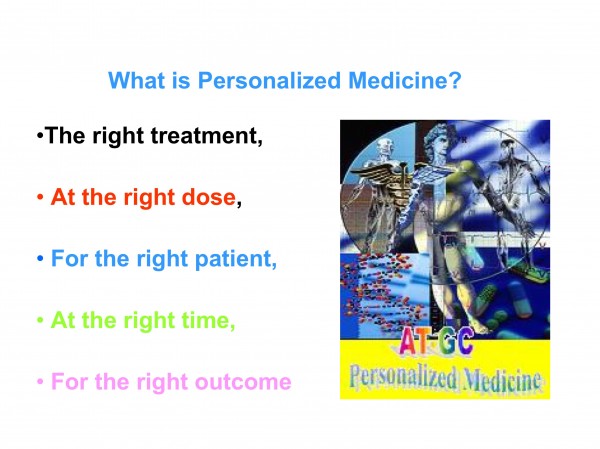
Figure 1: Definition of Personalised Medicine
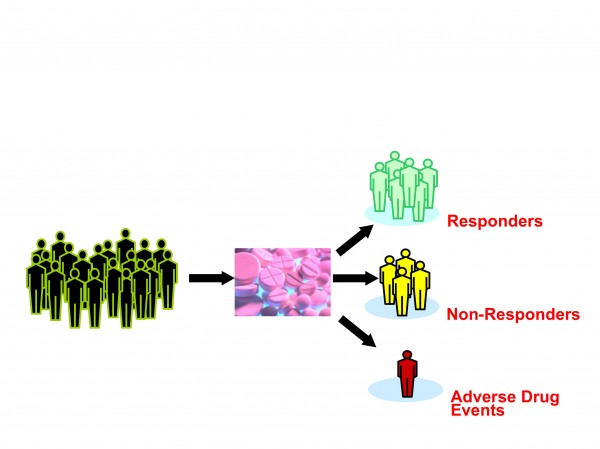
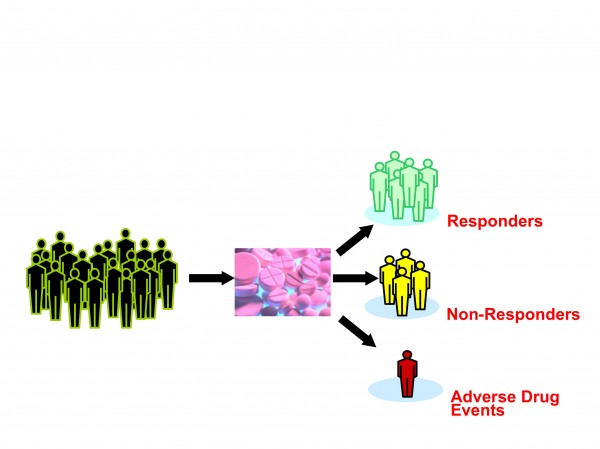
Figure 2: Current treatments take little account of human variation
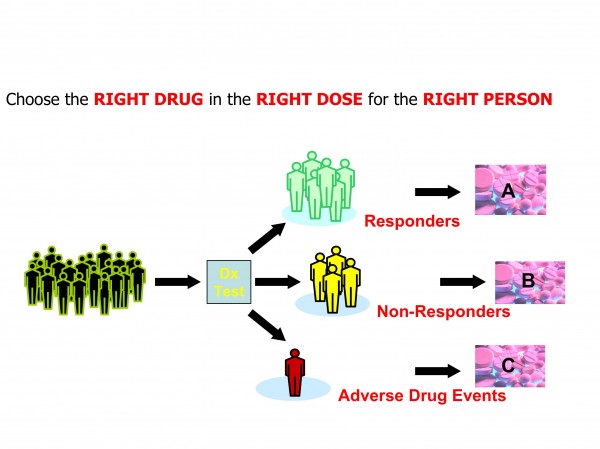
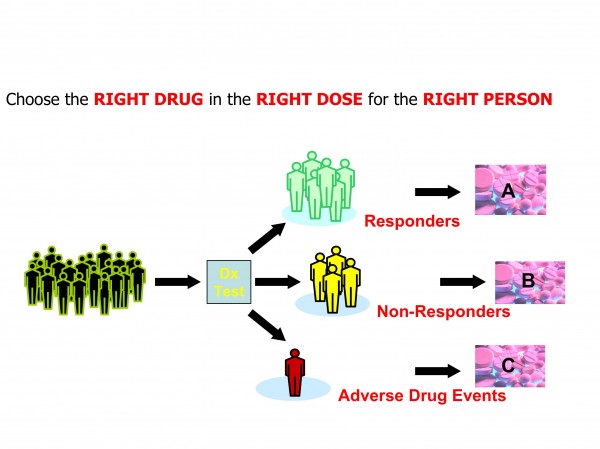
Figure 3: Personalised Medicine concepts foresee greater use of diagnostics in therapeutic decision making
Though this may sound a straight forward task, in actuality it poses major scientific challenges when one considers that there are approximately 3 billion base pairs in the human genome. For any two people, 99.9% of those nucleotides are the same. However, 0.1 % differences => 3 million nucleotides are different, causing inter-individual differences with different responses to the environment, food, drugs and susceptibility to diseases.
The real problem is how we analyse the 0.1 % differences that hold clues to the variations among human insusceptibility to diseases. Data on human genetic variation is useful to pinpoint genes responsible for the wide variability in people’s responses to different types of drugs; a field that is now referred to as pharmacogenomics.
Where does personalised medicine stand now?
As scientists learn more and more about individual genetic variations, the potential of personalised medicine grows. Although many of the first pharmacogenomic tests to check for genetic variations were most useful in alerting doctors about the likelihood of a severe adverse reaction to a drug, there are now a growing number of tests being developed to determine whether someone will respond to a particular medication. Thus, these tests may be able to predict if a drug will actually be helpful in improving the patient’s condition. However, despite the potential of personalised medicine, pharmacogenomics or proteomics testing are not widely available. The other stumbling block may be represented by the fact that some doctors may not yet be aware of their value or may not routinely use these tests in their medical practice.
Personalised medicine in drug development: evolving practices and biomarkers implementation
Sceptics argue that personalised medicine, and consequently biomarkers, are not a basis or stimulus for the next generation of new drugs, arguing that the term is just a novel word for basic tools to probe biology. Contrary to this school of thought, biomarkers are not new. Indeed, it is proven that biomarkers have long been part of good clinical preventive and diagnostic practice to measure biomarkers such as, blood pressure and serum cholesterol as potential risk factors for cardiovascular diseases. More controversial is the expansion into of the concept of biomarkers to improve the low success rate encountered in new drugs development, particularly in early clinical development and the use of biomarkers as a guide treatment decision maker based on genotype and phenotype.
Despite the challenges of accurately defining biomarker science and accessing relevant emerging technologies, the use and concept of biomarkers have had some recent successes in terms of regulatory approvals. Biomarker development forms one of the cornerstones of a new working paradigm in the pharmaceutical industry by increasing the importance of linking diagnostic technologies with the use of drugs. The position of the Food and Drug Administration (FDA) is rather clear on this; biomarkers are crucial to generate safe and efficacious drugs, they are also essential for deciding which patients should receive what treatment and when.
It appears that restructuring the development process in the pharmaceutical industry will also drive the development of biomarkers as diagnostic probes for clinical decisions and patients stratification (Figures 4 and 5).
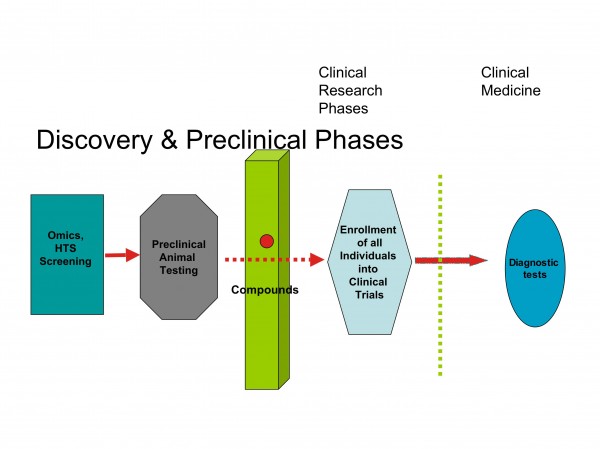
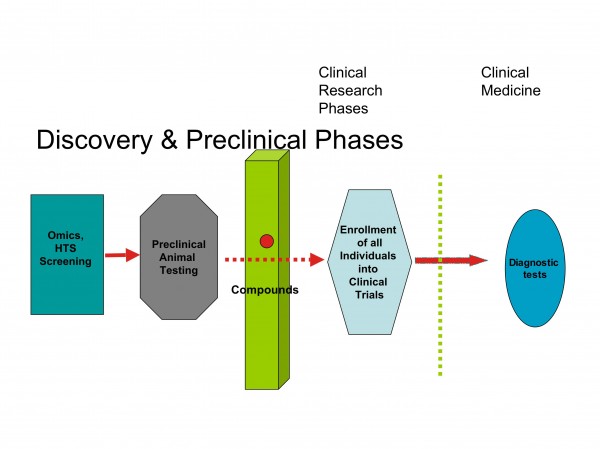
Figure 4: Barriers between discovery and clinical research
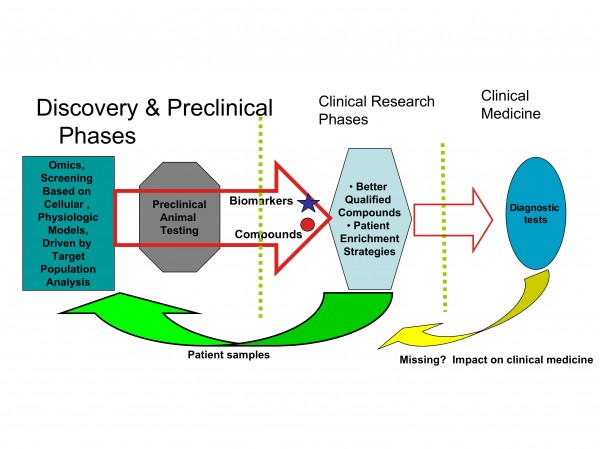
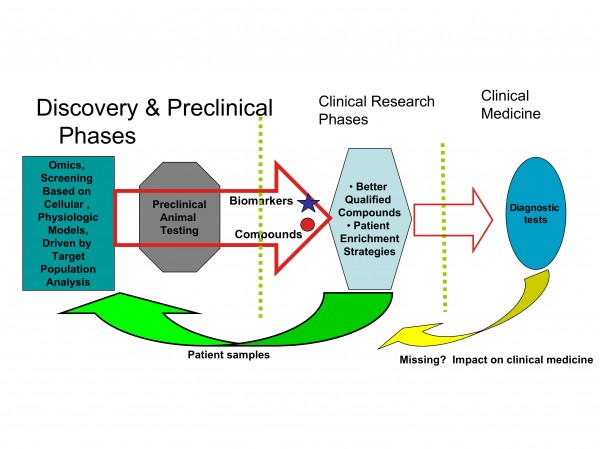
Figure 5: Biomarkers connect discovery and clinical research
Biomarker science is a field that is advancing rapidly; it could even be said that companies which fail to include a biomarker strategy in their development paradigm probably do so at their peril.
Personalised medicine: bridging biomarkers to clinical outcomes
The ideal situation for pharmaceutical and diagnostic companies would be to have the tools in order to establish and characterise who has a particular disease, which patient would benefit from which drug at what doses, and whether a drug will have adverse effects; compared to the current paradigm of waiting for years, and studying thousands of patients to determine the efficacy and safety of a new drug (Figure 6).
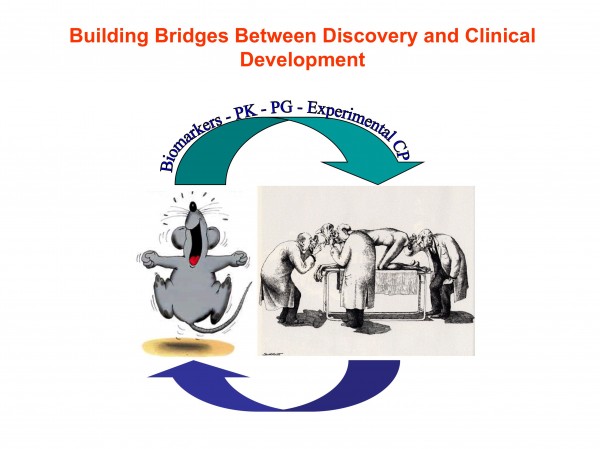
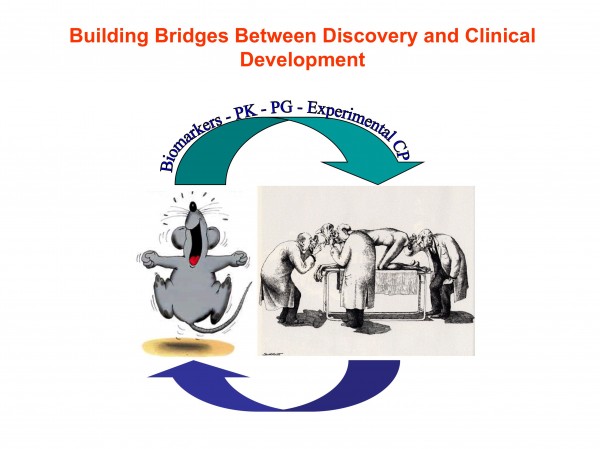
Figure 6: Translational Medicine/Personalised Medicine: building bridges between discovery and clinical development
To be considered predictive, a biomarker should, in most cases, satisfy two criteria:
- It must be associated with the biological mechanisms within a disease or treatment
- Must correlate statistically to clinical outcomes
Early clinical assessment of the potential value of biomarkers in decision making, either for product development or patient selection, is the focus of personalised medicine.
Deployment of a well designed biomarker strategy within the paradigm of personalised medicine provides a significant opportunity to enhance and streamline clinical development programs through accurate measurements of patient response, or predisposition to therapeutic intervention.
Biomarkers: the new paradigm
Aside from their use in diagnosis and prognosis, current applications of biomarkers in drug discovery and development include those that aid dosing and guide PK/PD indexes and those that help determine efficacy. The former are important in determining whether the dose is adequate but not excessive, particularly in early phase clinical studies. The latter serve as an important cost-effective surrogate function in lieu of traditional large-scale clinical studies with clinical endpoints.
Another important area well served by biomarkers, is drug safety and toxicity. Toxicity biomarkers may allow the exclusion of high risk patients before treatment is received, as well as monitoring for early patient withdrawal before any severe toxic effects occur (Figures 4 and 5).
Safety has implications at all stages of the drug development process and carries significant relevance for clinical trial design and regulatory approval. Since the purpose of pre-clinical toxicology testing is to assist in defining relevant safety concerns for the clinical trials, biomarkers developed and evaluated in these experiments can be adapted for conducting studies in healthy volunteers and patients.
Early identification of safety concerns, or indications that they will not be relevant, allows for more effective prioritisation and resource allocation. It is often necessary to work backwards from a phenotype (such as liver injury, vasculitis, renal damage) caused by an unknown mechanism to a specific parameter, or group of markers, that caused the injury. In contrast to many efficacy biomarkers, biomarkers of specific tissue damage can often be applicable to monitoring toxicity of a range of molecules acting via a variety of mechanisms, but on the same tissue or tissues.
Often, even more challenging to manage are the effects seen in pre-clinical species for which the clinical significance is unknown. Vasculitis has been an issue for a variety of therapeutic targets. In this instance, effects seen in animals may or may not be predictive of human risk, with the further complication that no reliable measures of the injury exist for monitoring in clinical trials. A biomarker panel capable of clearly monitoring for vascular injury and early changes would allow compounds to be safely tested in people, as well as having significant value in improving clinical practice related to vasculitis.
In many instances, it is possible to utilise an efficacy biomarker to predict undesirable effects, particularly in areas such as anticoagulants, Thrombin inhibitors, low molecular weight heparins, Factor Xa inhibitors and vitamin K antagonists are valuable therapeutic tools. Since at high doses most of these molecules exhibit only minimal toxicity, other than those mediated through the same pathways as their pharmacologic methods of action (such as, bruising or internal bleeding), the same assays that predict their activity can be used to assess toxicity. Tests such as ecarin times, activated partial thromboplastin time, prothrombin time and Factor Xa activity all represent useful safety and efficacy biomarkers. Since these assays show considerable translatability across species and are already within the repertoire of hospital laboratories, their acceptance by clinicians and regulatory agencies is enhanced.
Biomarkers and decision making
Historically, biomarkers referred to analytes in biological samples, or any measurement that predicted a disease state or response to a specific drug therapy. Biomarkers are valuable drug development tools that provide more accurate/complete information regarding drug performance and disease progression.
Developments in genomics and proteomics have renewed the interest in biomarkers as useful clinical tools. While the speed of development for these technologies has been impressive, the interpretation of data has not yet reached maturity. The time required to characterise and validate new biomarkers often results in them lagging behind other rapidly evolving technologies.
For specific biomarker panels, there is a risk that by the time a biomarker or a panel of biomarkers is validated it may be too late in the drug development process to be of value in decision making. Nevertheless, biomarkers constitute a rational approach, which, at optimal level, reflect the biology of the disease and the effect upon the drug candidate. Appropriate incorporation of biomarkers in drug development strategies can enable the concept of ’fail fast, fail early;’ facilitating the choice of an appropriate critical path toward approval and even differentiation between approved products in a competitive market place. The challenge is therefore to identify relevant biomarkers early enough to implement them for ‘go, no-go’ decisions at critical stages throughout the drug development process (Figure 7).
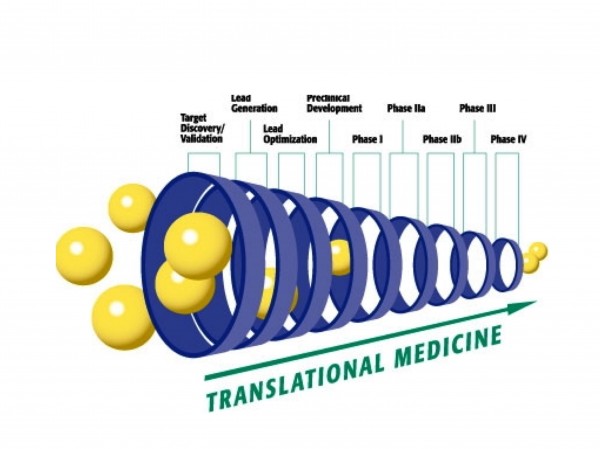
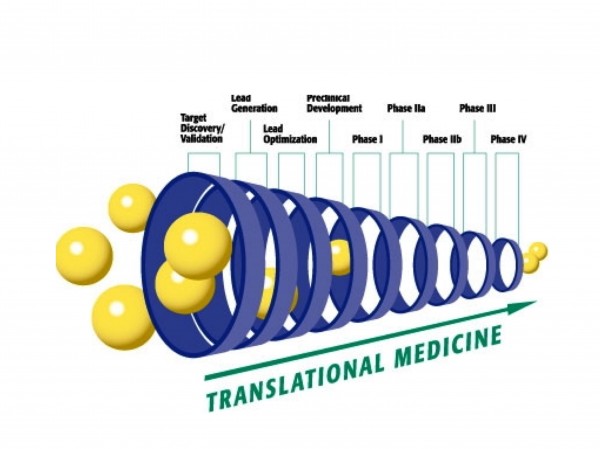
Figure 7: Translation medicine and biomarkers: affecting the different phases of drug development
However, there is a wide array of considerations that need to be balanced against each other and kept in mind when selecting a biomarker so as to ensure the test developed will meet the intended goal and actually be used. Among the multiple areas that need to be defined are: the nature of the biomarker’s use (activity, efficacy, safety, surrogate), throughput, capacity, turnaround time requirements; reproducibility, sources of physiologic variation, and sensitivity; stability of parameter and compatibility with the study design; timeframe in which an appropriate method needs to be developed and defining the ways in which the biomarker adds value.
Biomarkers play a key role in predicting the failure rate in drug development
Biomarkers are emerging as an essential tool to boost efficiency in the field of drug discovery. The relatively high failure rate (50% – 85%) of new drugs in Phase II due to lack of activity/efficacy, translates into significant opportunity for improvement if these same molecules could be identified pre-clinically or in Phase I clinical trials. A lot of compounds also fail during the clinical trials; these failures are predominantly related to absorption, distribution, metabolism, excretion (ADME), safety and toxicity issues.
Biomarkers increase productivity by identifying potential drug failures at an early stage “fail fast, fail early”, thereby saving time and money in the long-term.
Biomarkers in pre-clinical development
Drug development was traditionally described as pre-clinical development followed by clinical Phases I through IV. Today, drug development is seen as an integral data driven process in which the single phases overlap and more importantly, use all information gained to create an optimal design for the next steps1 (Figures 4 and 5). In pre-clinical development, biomarkers are an essential element of the processes used to optimise the science of drug development. These processes include the use of a number of modern technologies, such as functional genomics, proteomics, imaging, modeling, metabonomics, computational methods, informatics and modern analytical technologies.
Biomarkers in clinical development
In early clinical studies, the use of suitable biomarkers should be considered in demonstrating proof of concept and identifying appropriate dose regimens for efficacy studies, doing this and learning which sub-populations are most likely to benefit from the new treatment, may help in the planning of later efficacy studies. Although the approach may seem straightforward, the use of biomarkers carries with it a number of practical pitfalls.
It should be noted that any clinical observation in a given subject might serve a different purpose and each purpose can have implications for the way these characteristics are used, documented, validated and interpreted. In clinical practice, for example, blood pressure is measured to assess the individual risk for a given patient and to decide upon that patients further diagnostic and therapeutic management. The same characteristic may be determined in epidemiological research. Finally, blood pressure may be used as an outcome measure for a company’s decision on a specific research project. The same measure may become a valid surrogate endpoint and an essential element in the regulatory review process to be used in the decision making process for marketing approval of the new drug.
Selecting biomarkers
A major challenge in the selection of a biomarker is to characterise it as valid. A validated biomarker may be defined as one that is widely accepted as having physiological, toxicological, pharmacological, or clinical significance before it is used in drug development, thus justifying its use in the regulatory process. A drug may be given accelerated approval status based on the expression pattern of certain validated biomarker(s), without actually meeting a ‘gold standard’ for primary endpoints in a large-scale clinical trial. Alternatively, clinical studies may be curtailed because the drug does not affect a specific biomarker, although it may have proven efficacious if typical late phase clinical trials had been conducted.
Pre-clinical validation of biomarkers would be ideal since it is faster and less expensive than clinical validation. However, this is often unrealistic given the inconsistent results typically encountered between animal models and the clinical diseases they simulate.
Assaying and assessing biomarkers
Numerous biochemical and physical read outs from various body fluids or tissues are currently being explored. However, the technologies utilised to assay many of these biomarkers can be cumbersome (metabolites), technically challenging (proteins) or expensive (imaging).
Interpreting biomarkers outcomes
Biomarkers play an especially critical role during Phase IIA. To correctly interpret the outcome of clinical studies in which the primary outcome variable is a biomarker, those substitutes for the relevant clinical outcome; scientists must consider the complex relationship between the medical condition, the specific biomarker and the clinical outcome.
AIDS is a straightforward example; CD4 counts and viral load are two of the very few validated biomarkers that substitute the relevant clinical outcome. Indeed, those are associated with the disease status, prognosis and therapeutic intervention.
However, the straightforward association between a disease, a biomarker and the clinical outcome oversimplifies the underlying pathophysiology of the disease. Thus, a disease is generally associated with several clinical manifestations, which may or may not serve as biomarkers and which may be associated with the relevant clinical outcome. The possibility that the clinical outcome is time dependent must also be taken into account when interpreting the outcome of studies using biomarkers. It can be concluded that biomarkers and clinical endpoints are not completely dependent on one another, but that there is a fairly well-defined relationship between the two.
High costs and duration of clinical trials drives biomarker research
There is growing concern in the field of drug discovery regarding cost and duration of clinical trials reaching magnified proportions. A new drug is estimated to cost anywhere between $800 million and $1,700 million and is expected to take anywhere between 7 and 12 years to be approved and launched.
Within the pharmaceutical industry, biomarkers are seen as one of the most promising tools for reducing the cost of developing drugs and ensuring that the appropriate patient populations are treated. The goal of a biomarker’s use is to identify the right projects to devote scarce resources at the earliest possible stage and to increase productivity by increasing the number of new drugs that achieve registration. Proper use of these tests can improve the quality and quantity of mechanistic information obtained at each step of the drug development process, allow better understanding of other results and factors relevant to their interpretation, plus provide clearer indications of a molecules activity or potential safety liabilities. A biomarker can also differentiate a new drug from other products already on the market. Examples of biomarkers include cholesterol as an indicator of heart disease risk, alanine aminotransferase to monitor liver injury, changes in circulating lymphocytes to assess effects on immune function and magnetic resonance imaging (MRI) of joints to characterise arthritis. Clearly, there is a wide spectrum of technologies encompassing biomarkers, with individuals from many specialties involved in the field with varying degrees of success.
Biomarkers: a new tool to save the pharmaceutical industry time and money
Pharmaceutical companies face the challenge of moving new compounds to market as rapidly as possible. During development, they attempt to recognise and minimise the cost of products that cannot pass the registration process because of toxicity issues or lack of efficacy. However, the past few decades have demonstrated unprecedented silences and culture divides between clinical and discovery research both in the academic or pharmaceutical industries. In both settings, basic and clinical research coexisted side by side but interacted only indirectly. In the past few years however, the situation has begun to change and dedicated efforts to bridge the gap between bench and bedside have been called.
Important gaps between discovery and development continue to be a defining feature of the pharmaceutical industry’s culture. In discovery, the emphasis is on innovation, while in development it is on speed and process. In both cases, the methods are very different and communication is often ineffective. For many decades, this process has served the industry. Indeed, until recently, no one felt the need for translational medicine to bridge this gap.
Most drug discovery and development efforts involved making chemical improvements on existing compounds directed against a little less than 500 well-validated targets that had already proven their efficacy in the clinic2; a slow, steady flow of development candidates could be handled efficiently.
This balance was disrupted by the adoption of new technologies by discovery organisations. Combinatorial chemistry, high throughput screening and the availability of the human genome sequence had several major consequences; the most significant was that they dramatically increased the number of targets for discovery3. The number of candidates available for clinical development increased several fold in the past few years. These technologies opened the door to novel targets that never before been pharmacologically tested in humans. No matter how well these targets responded in animal models, there was no longer any assurance they would be significant in humans; detailed studies of failure rates bear out these concerns. The break point in the pipeline has shifted to the transition between discovery and development. The highest rate of failure (approximately 50% between 1980 and 1992) is in Phase II proof-of-concept studies4. This failure rate has almost doubled in the last decade.
As it may have been predicted, most of the new compounds that work very well in laboratory animals do not work in humans. Cost-benefit analyses of existing development protocols no longer apply. Contrary to this, Phase III failure rates have remained fairly static in the last decade. Reducing failure rates in Phase II proof-of-concept studies is now the primary goal of the pharmaceutical industry.
Breaking down the silence
Basic research and clinical practice of medicine is often carried out in with very little communication. Drugs were developed independently of the clinical needs and often the first contact with the clinic was to test for safety and efficacy in clinical trials. The recent move towards personalised medicine and translational medicine is focused on removing this silence and stimulating the exchange of ideas and understanding the possibilities of basic research in clinical practice; true ’bench to beside’ research.
One of the most tangible problems that scientists have faced in recent years is locating biomarkers that are predictive or ‘translate well’ from animal models to humans. For example, inhibiting an enzyme in animal models can have a dramatic effect in the animal, whereas inhibiting the same enzyme in a human could have very limited or negligent clinical impact. The reasons for the lack of correlation are many and varied:
- The therapy can change a biomarker but be irrelevant to the disease
- The biomarker may not reflect clinically important effects of the treatment
- In humans a drug may work through several different pathways not reflective in animal models. Thus, the fundamental challenge for a biomarker is not to unravel sophisticated pathogenetic mechanisms, but rather to understand how the biomarker reflects the biology of the disease. A drug should treat a disease, not a biomarker.
If the biology of the disease is not understood; it is difficult to ascertain which biomarker should be chosen. Appropriate biomarker evolution requires close dialogue between biomarker scientists and practicing physicians, this can be accomplished under the banner of personalised medicine.
Putting biomarkers to work and the role of contract research organisations
One must consider the time and money spent or saved versus the confidence gained from the use of biomarkers in ongoing drug development; there is a significant opportunity to enhance and streamline clinical development programs through accurate measurements of patient response, or predisposition to therapeutic intervention. Co-development of biomarkers during the development cycle of drug candidates is a synergistic way in which to leverage knowledge of biological mechanisms. Early clinical trials of drug candidates provide a good environment for simultaneously evaluating candidate biomarkers. Timely biomarker data provides more accurate information regarding drug performance and leads to better drug development decisions, particularly early on in clinical development.
The interest of diagnostic companies in marketing rights to a specific biomarker will be influenced by factors such as market share and company profile. There are few, if any, pharmaceutical or biopharmaceutical companies that have the depth or breadth of expertise and resources to develop, support and market diagnostic kits. Consequently, partnering between pharmaceutical and diagnostic companies is becoming a common practice. This trend will increase in the future with the evolving concept of personalised medicine.
Designing new drugs
Data on human genetic variation can be used to pinpoint genes responsible for the wide variability in people’s responses to many common drugs. Traditionally, basic research and the clinical practice of medicine has been secluded in poor communication; in the past, drugs were developed independently of the clini, and often ‘thrown over the fence’ to be tested for safety and efficacy in clinical trials. The recent move towards personalised medicine is focused on the removal of this silence, encouraging both the exchange of ideas and understanding the potential of basic research in clinical practice and neglected medical needs. The increasing demand for personalised medicine, prevention trials dependent on markers of disease progression and drug development strategies targeting complex biological systems are powerful drivers for increased use of these multi-factorial biomarkers in all therapeutic areas. However, interpretation of multi-factorial biomarkers in development decisions remains a challenge and the full potential of their role has yet to be realised. Biomarkers and their assays are an integral part of personalised medicine. These biomarker assays often developed early on, at the pre-clinical stage if appropriate, can be the key to integrating a program from pre-clinical/early clinical studies to proof-of-concept studies in patients and beyond.
Conclusion
Personalised medicine has the potential to be useful in early clinical drug development; it can assist in compound selection as well as the demonstration of early clinical concept proof. However, the real issue is the time required to understand and characterise new biomarkers. By the time a personalised medicine strategy and biomarker/panel of biomarkers gets validated, it can be too late in the process for them to be influential in decision making. The real question is, does personalised medicine change the way we develop drugs and practice medicine? Do biomarkers really measure something that is relevant to the disease or drug and can we discern their use in decision making promptly?
References
1. Lesko, L.J.; Rowland, M.; Peck,C.C.; and Blaschke, T. J Clin Pharm. Vol 40 pp803-814 (2000).
2. Drews, J. In Quest of Tomorrow’s Medicines, Springer, New York (1998).
3. Archer, R. Nature Biotechnology, vol. 17, p. 834 (1999).
4. Dimasi, J.A Clin. Pharmacol. Ther., vol. 69, pp. 297-307 (2001).
Claudio Carini, F. M.D., Ph.D., FRCPath, F.
VP of TM at MDS Pharma
Dr. Carini was former Global Head of Translational Medicine and Head of The Biomarker Alliance at MDS Pharma. Prior to joining MDS, he served as Global Head of Biomarkers at Hoffman La Roche, and before that as Global Director and Therapeutic Area Head of Translational Medicine at Novartis. He has developed several compounds in the anti-inflammatory arena for different indications, and has extensive experience in interacting with regulatory agencies. He has successfully implemented translational research to reduce the length and cost of clinical development, and has managed and coached technical and clinical staff through all phases of clinical development from inception to completion.
Candida Fratazzi M.D.
Senior Director, Altus Pharma
Dr. Fratazzi is the Senior Medical Director and Clinical Project Leader at Altus Pharmaceuticals. Prior to joining Altus, she served as Senior Medical Director and Team Leader at Shire Pharmaceuticals. She identified strategic clinical developments for several biologics and small molecules compounds to treat viral/bacterial, lysosomal storage and inflammatory diseases. She has extensive experience in interacting with different agencies such as FDA, EMEA, Health Canada, AMNAT, and MOH in Israel. She has successfully implemented several international clinical studies by mastering the art of multi-cultural management.