Status and challenges in structure-based drug discovery for G protein-coupled receptors
Posted: 13 December 2011 |
The central location of G protein-coupled receptors (GPCRs) at the interface between the interior and exterior of cells, as well as their key role in signalling events, make GPCRs a prominent class of pharmaceutical targets. To date, approximately 40 per cent of known drugs are thought to act on GPCRs either directly or indirectly. GPCRs are for the most part inaccessible to structural determination due to difficulties to express, purify and crystallise them; however, progress of structure determination has led to seven new structures in the last decade. This number is still insufficient to conduct structure-based drug discovery on all available targets. Computational modelling is therefore a very useful surrogate and in this paper I discuss the reliability of atomistic three-dimensional models that are obtained through molecular modelling in light of the GPCRdock 2008 and 2010 competitions organised by the Scripps Institute. G protein coupled receptors (GPCRs) are key proteins involved in signalling and as such are prominent drug targets. Ligands that bind to GPCRs include small aminergic neuro – transmitters or hormones such as noradrenaline and adrenaline, dopamine, histamine, small peptides, nucleic acids, lipids or even opsins that contain light-reactive retinal chromophores. Altogether, in the human genome project, about 390 non-olfactory GPCRs have been identified; of which about 100 are orphan proteins without an identified ligand or cellular function…
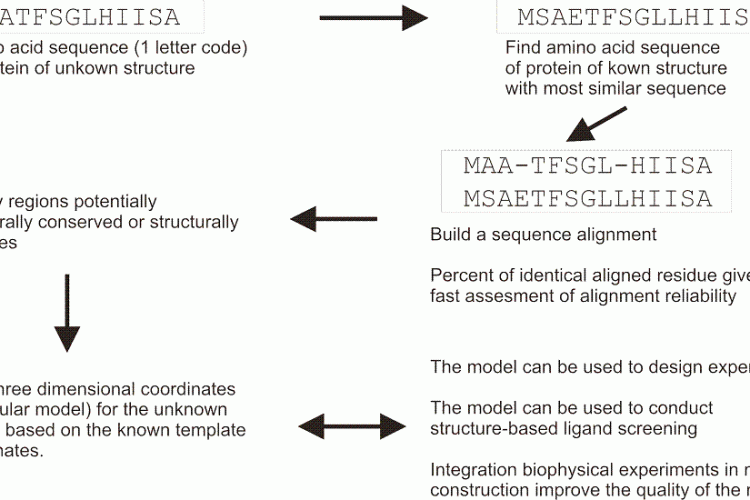
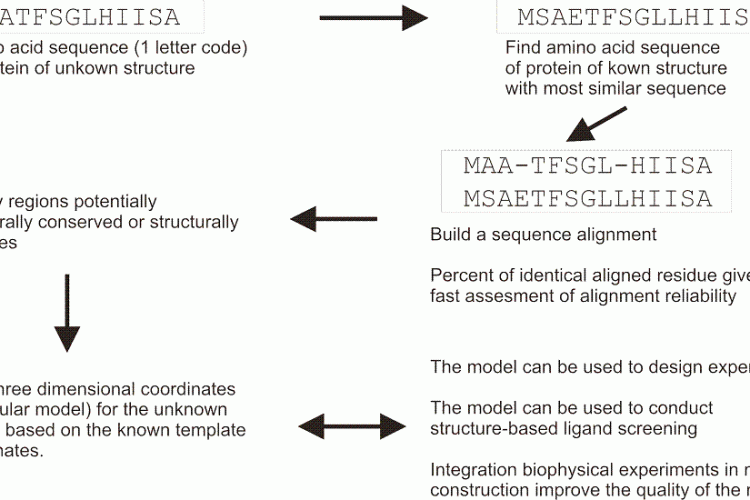
FIGURE 1 Schematic overview of a typical homology modelling procedure that is used to build threedimensional coordinates for a protein of unknown structure
The central location of G protein-coupled receptors (GPCRs) at the interface between the interior and exterior of cells, as well as their key role in signalling events, make GPCRs a prominent class of pharmaceutical targets. To date, approximately 40 per cent of known drugs are thought to act on GPCRs either directly or indirectly. GPCRs are for the most part inaccessible to structural determination due to difficulties to express, purify and crystallise them; however, progress of structure determination has led to seven new structures in the last decade. This number is still insufficient to conduct structure-based drug discovery on all available targets. Computational modelling is therefore a very useful surrogate and in this paper I discuss the reliability of atomistic three-dimensional models that are obtained through molecular modelling in light of the GPCRdock 2008 and 2010 competitions organised by the Scripps Institute.
G protein coupled receptors (GPCRs) are key proteins involved in signalling and as such are prominent drug targets1. Ligands that bind to GPCRs include small aminergic neuro – transmitters or hormones such as noradrenaline and adrenaline, dopamine, histamine, small peptides, nucleic acids, lipids or even opsins that contain light-reactive retinal chromophores. Altogether, in the human genome project, about 390 non-olfactory GPCRs have been identified; of which about 100 are orphan proteins without an identified ligand or cellular function.
In cells, GPCRs are embedded in lipidic membranes, leading these proteins to adopt a fold composed of seven transmembrane helices, hence the names 7TM or serpentine receptors. GPCRs are critical in mediating signalling events, where ligand binding to the receptor leads to a conformational switch in its structure, activation of an intracellular G-protein and triggering of second messenger pathways. This key location at the interface between inside and outside the cells makes GPCRs validated or emerging drug targets. To date, approximately 40 per cent of marketed drugs are thought to target GPCRs directly or indirectly2 and individual GPCRs are often seen as useful targets for several therapeutic areas. For example, α2-adrenoceptors are thought to be useful targets for the treatment of elevated blood pressure and intraocular pressure, in alleviating withdrawal symptoms from opioid and in alcohol abuse, as well as anesthetic adjuvants. It is therefore of no surprise that GPCRs are of key interest to the pharmaceutical industry.
Ligand-based methodologies in drug discovery relate to the methods that use as starting material the structure of a known ligand and look for more potent or chemically accessible derivatives. Drugs acting at least partially via GPCRs, such as morphine, ephedrine or mescaline, have been documented for a long time. Endogenous chemicals able to activate GPCRs, such as the chromophore 11-cis-retinal or the small molecules such as adrenaline were identified at the start of the 19th century but their mode of action via GPCRs was clarified only much later. In addition to chance observation, drug molecules have been derived from the chemical structure of known molecules, for example the β-blocker propanolol and the antihistaminic cimetidine, which were synthesised in the 1960’s by Sir James Black, recipient of the Nobel prize in 1988 for that work.
A classical approach in modern ligandbased design is to use a pharmacophoric description, or chemical substructures, as starting points to screen in silico commercial or private compound libraries. The last two decades have seen the development of Selective Optimisation of Side Activities (SOSA) that aims at finding new targets for old drugs, and this strategy has shown itself to be very useful with GPCRs that hold many side activities3. More recently, fragment based methodologies have been used for GPCRs too4. A potential drawback of ligand-based methods is that they usually suggest molecules that resemble the initial chemical scaffold.
Structure-based GPCR drug discovery
A more ambitious strategy in drug design is to use information from the atomistic threedimensional atomic structure of the target protein. This strategy requires knowledge of the atomic coordinates for the receptor. In terms of drug discovery, receptor structure-based drug design has its own advantage since it is more likely to result in an entirely novel chemical scaffold than ligand-based methods.
A common description of the molecular mechanism of ligand binding is given by the lock-and-key classical analogy, although a more modern conception is the description of a handin- glove that better represents the flexibility of the interaction partners. A large number of GPCR ligands bind within a pocket located within the plane of the helical transmembrane bundle5. This binding pocket, also called binding cavity, is faced by amino acids that mirror the physicochemical properties of the ligand molecules, allowing an optimal network of weak molecular interactions to take place. The event of ligand binding is complicated to study, due for example to errors in model structures, to the dynamics of the receptor, to water molecules that associate to both ligand and receptor, bridging polar groups of ligand and generating salvation / desolvation costs that are difficult to measure accurately.
The key step to conduct structure-based drug discovery is therefore to obtain the three dimensional coordinates for protein itself. While the first GPCR, rhodopsin, was sequenced in 1975, followed by the adrenergic receptor in the 1980s, it is only in the last decade that reliable structural information has become available for this family. GPCRs are embedded in the cell membrane and have proven themselves to be very resilient to X-ray crystallography, being very difficult to express, solubilise, and crystallise. Rhodopsin, expressed in the eye at high concentration naturally, was the first GPCR structure to be solved at atomic resolution in 20006, although the 3D electronic density of the helices had been determined by cryo-electron microscopy in the 1990s. The human β2-adrenoceptor7 and the turkey β1-adrenoceptor8, the dopamine 3 receptor9, the chemokine CXCR410, and the histamine 1 receptor11 were solved following major break – throughs in the field of X-ray crystallography: ligand-affinity chromatography for purification, robotic systems for microcrystallisation, or e.g. stabilisation by fusion with the T4 lyzosyme protein in place of the third intracellular12,13.
In the absence of crystal structures, molecular models are a widely used surrogate to conduct structure-based drug discovery. Molecular modelling is inexpensive and for GPCRs often the only accessible strategy to gain an atomistic understanding in the ligand binding properties. Molecular modelling aims both to predict the three dimensional structure of proteins and, with molecular docking simulations, to predict the energetically optimal virtual fit of a ligand inside a binding pocket. Docking simulations are also used for prioritisation of compound libraries, i.e. to rank compounds, through virtual screening experiments14.
Methods to build three-dimensional atomic models of GPCRs
Amino acid sequences have been the first and most accessible type of data available for GPCRs, and amino acid sequences are imprinted with information about the three-dimensional structure. Early methods have accurately predicted the seven helical structure based on hydropathy plots, hydrophobic moment plots have resolved for most part the rotational orientation of the helices, while receptor orientation has been successfully predicted using the positive-inside rule. The simple topological models derived from these methods have been very useful to help predict which amino acids are forming the binding pocket, which in turn were used to design mutated receptors to validate the predictions.
The first glimpse of the seven-trans – membrane helical GPCR structure came from cryo-EM maps, which were used to fit experimental data and to build low resolution 3D models in combination with sequencebased prediction methods. Bacteriorhodopsin, a bacterial proton pump predicted to have seven helices and covalently bound with a retinal chromophore, rhodopsin, was first thought to share similarities with known GPCRs and was used early on for structural modelling15. Nonetheless, only the threedimensional structure of bovine rhodopsin really opened new avenues in the field, serving as a basis for a wealth of studies based on homology modelling.
Homology modelling is based on the hypothesis that related proteins share a common fold. In homology modelling (Figure 1), a known protein structure is used as a structural template to build the threedimensional coordinates of an unknown one. A pairwise sequence alignment is used to define the equivalent amino acids. The alignment may allow the user to identify structurally conserved regions, well aligned, and structurally variables regions, often poorly aligned. The protein fold is shared within structurally conserved regions, but the structurally variables regions needs to be modelled independently of the structural template. A commonly used benchmark to represent the evolutionary closeness and therefore the accuracy of the model is a percentage of sequence identity: two random sequences have a sequence identity at most of 15 per cent, and GPCRs share usually about 18-20 per cent identical residues in their transmembrane regions, while receptors for example among the amine family will share 40 per cent. In GPCR modelling to date, the main computational challenge is to find and allow productive deviations from the atomic coordinates inside the structurally conserved regions, as well as in the independent modelling of the structurally variable regions.
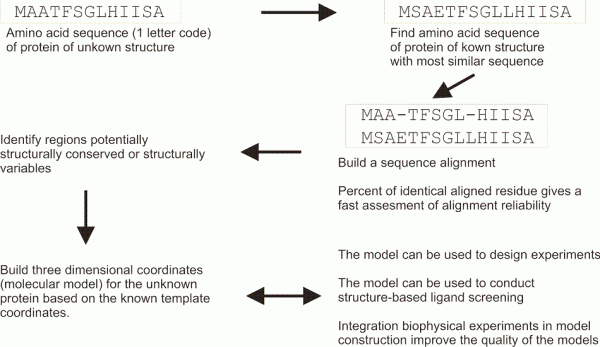
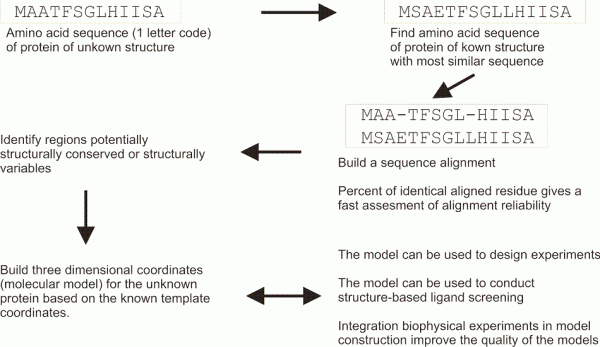
FIGURE 1 Schematic overview of a typical homology modelling procedure that is used to build threedimensional coordinates for a protein of unknown structure
State-of-the-art in GPCR complexes modelling: GPCRdock 2008 and GPCRdock 2010
The Scripps Institute has recently organised benchmarking competitions, where the participating groups were asked before the release of the three-dimensional coordinates to build structural models of the adenosine 2A receptor (GPCRdock 2008)16, and of the dopamine 3 and the chemokine CXCR4 receptors (GPCRdock 2010)17, as well as their interaction with ligands. A maximum of one month was given to conduct the modelling study and five to ten models at maximum could be submitted for each group, after that the X-ray structure was released. This type of experiment presents a very accurate evaluation of the pitfalls and of the successes achievable in the field.
A major finding of these studies is that homology modelling followed by docking can be relatively accurate when structure and template share above 35 per cent of identical amino acid, but human knowledge plays an important role: only the best research groups were able to recreate atomic resolution complexes in the most accessible experiment (Figure 2). Generally, the experiment confirmed that the transmembrane helices are nearly always well modelled but for some specific local deviations such as kinks. On the other hand, it is not possible to date to construct accurate models of the loop region of the receptors in a robust and reliable way. Alternatives to homology modelling, for example MemSTRUK, TASSER, GPCRfold, were shown to be in constant improvement, however they did not fare as well as homology modelling. Docking the ligand appeared to be a major challenge. In this type of double-blind study, as in real cases, if the modelling of the receptor structure is wrong, for example if the binding site has been partly obstructed by loops, it impacts the simulation by preventing the successful docking of ligands.
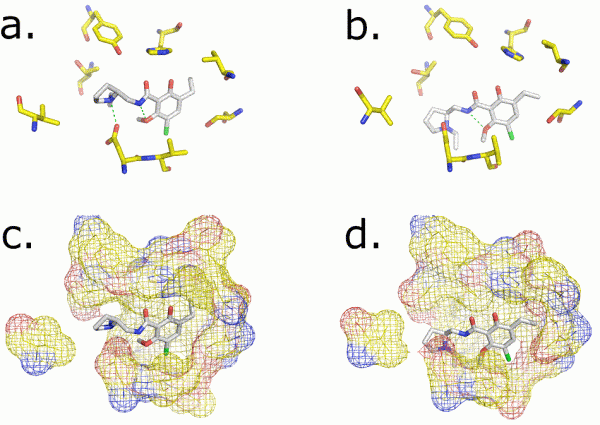
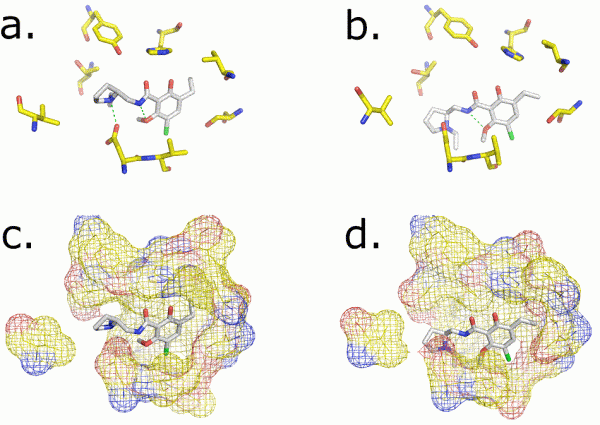
FIGURE 2 Near-atomic resolution modeling of Eticlopride binding to the Dopamine 3 receptor. Selected amino acids lining the binding pocket and their surfaces are shown for the crystal structure (a and c) and for the computational model proposed by myself (b and d) to the GPCRdock 2010 competition. Two key interactions, a hydrogen-bond and salt bridge, are indicated by a green dashed line
The GPCRdock 2010 proposed three targets: the dopamine 3 receptor, the chemokine CXCR4 receptor in complex with a peptide and a small molecule. The dopamine 3 receptor, which is a relatively close relative to the β-adrenoceptor, was well modelled in complex with eticlopride by about one third of the groups. Using experimental information and a sound chemical knowledge appeared key to success. The second target proved itself very challenging and although some groups found the general location of the peptide on the receptor, close to the extracellular surface, no group managed to get an atomistic picture of the molecular interactions. In GPCRdock 2008, modelling of the adenosine 2A receptor with the ligand was also very challenging, with only one group succeeding in building a reasonable model. The successful group had long-lasting experience in working with the adenosine 2A receptor and the SAR series of ligand, showing a clear connection between human knowledge and the ability to build successful GPCR-ligand complexes.
Summary
In summary, computer simulations have proven to be invaluable tools to provide structural information on GPCRs and their interaction with ligands. This type of method still has a brilliant future due to the high pharmaceutical importance of these receptors as drug targets and to the relative inaccessibility of GPCRS to structure determination – although the last decade has seen many improvements in that field. The helical region of GPCRs is usually conserved and well modelled. The reliability of the molecular models can be predicted using simple metrics such as sequence identity. For distant receptors, accurate atomistic modelling is not accessible although these models can be useful to design experiments. For receptor sharing more 35 per cent sequence identity to template, recent blind modelling competitions have shown that it is possible to obtain reliable atomistic models, but only when a wealth of pharmacological and biophysical data is integrated in the molecular models.
About the Author
Henri Xhaard is principal investigator at the Centre for Drug Research, Faculty of Pharmacy, University of Helsinki, Finland where he leads the Computational Drug Discovery group. He received his PhD in 2006 from the University of Åbo Akademi, Finland, after a MSc in molecular biophysics conducted at the University Paris VI. His main expertise is computational modelling of G protein-coupled receptors and he has been ranked among the world top groups in the recent GPCRdock 2010 competition organised by the Scripps institute. The current research of his group includes, in addition to computational drug discovery, pharmacokinetic predictions using QSAR modelling, and development of computational tools to accelerate drug discovery.
References
1. Lundstrom KH, Chiu ML, editors. G protein-coupled receptors in drug discovery. CRC Press; 2006
2. Overington JP, Al-Lazikani B., Hopkins AL. How many drug targets are there. Nature Reviews Drug Discovery 2006; 5:993-996
3. Allen JA, Roth BL. Strategies to discover unexpected targets for drugs active at G protein-coupled receptors. Annu Rev Pharmacol Toxicol. 2011; 51:117-144
4. Congreve M, Langmead C, Marshall FH. The use of GPCR structures in drug design. Adv Pharmacol. 2011;62:1-36
5. Mouillac B, Chini B, Balestre MN, Elands J, Trumpp- Kallmeyer S, Hoflack J, Hibert M, Jard S, Barberis C. The binding site of neuropeptide vasopressin V1a receptor. Evidence for a major localization within transmembrane regions J Biol Chem. 1995;270:25771-7.
6. Palczewski K, Kumasaka T, Hori T, Behnke C, Motoshima H, Fox B, Trong I, Teller D, Okada T, Stenkamp R, Yamamoto M, Miyano M. Crystal structure of rhodopsin: A G protein-coupled receptor. Science 2000. 289:739-745
7. Cherezov V, Rosenbaum DM, Hanson MA, Rasmussen SG, Thian FS, Kobilka TS, Choi HJ, Kuhn P, Weis WI, Kobilka BK, Stevens RC. High-resolution crystal structure of an engineered human beta2-adrenergic G protein-coupled receptor. Science.200, 318, 1258-65
8. Warne T, Serrano-Vega MJ, Baker JG, Moukhametzianov R, Edwards PC, Henderson R, Leslie AG, Tate CG, Schertler GF.. Structure of a beta1-adrenergic Gprotein- coupled receptor. Nature. 2008, 454, 486-91
9. Chien EY, Liu W, Zhao Q, Katritch V, Han GW, Hanson MA, Shi L, Newman AH, Javitch JA, Cherezov V, Stevens RC. Structure of the human dopamine D3 receptor in complex with a D2/D3 selective antagonist. Science. 2010;330:1091-5
10. Wu B, Chien EY, Mol CD, Fenalti G, Liu W, Katritch V, Abagyan R, Brooun A, Wells P, Bi FC, Hamel DJ, Kuhn P, Handel TM, Cherezov V, Stevens RC. Structures of the CXCR4 chemokine GPCR with smallmolecule and cyclic peptide antagonists. Science.010;330:1066-71
11. Shimamura T, Shiroishi M, Weyand S, Tsujimoto H, Winter G, Katritch V, Abagyan R, Cherezov V, Liu W, Han GW, Kobayashi T, Stevens RC, Iwata S. Structure of the human histamine H1 receptor complex with doxepin. Nature. 2011, 475:65-70
12. Landau EM, Pebay-Peroula E, Neutze R. Structural and mechanistic insight from high resolution structures of archeal rhodopsin. FEBS letter 2003; 555: 51-56
13. Cherezov V. Peddi A, Muthusubramanian L, Zheng YF, Caffrey M. A robotic system for crystallizing membrane and soluble proteins in lipidic mesophases. Acta Crystallogr 2004: D60: 1795-1807
14. Bissantz C, Bernard P, Hibert M, Rognan D. Proteinbased virtual screening of chemical databases. II. Are homology models of G-Protein Coupled Receptors suitable targets? Proteins. 2003;50:5-25
15. Trumpp-Kallmeyer S, Hoflack J, Bruinvels A, Hibert M. Modelling of G-protein-coupled receptors: application to dopamine, adrenaline, serotonin, acetylcholine, and mammalian opsin receptors. J Med Chem. 1992;35:3448-62
16. Michino M, Abola E; GPCR Dock 2008 participants, Brooks CL 3rd, Dixon JS, Moult J, Stevens RC. Community-wide assessment of GPCR structure modelling and ligand docking: GPCR Dock 2008. Nat Rev Drug Discov. 2009:455-63
17. Kufareva I, Rueda M, Katritch V, Stevens RC, Abagyan R; GPCR Dock 2010 participants. Status of GPCR modelling and docking as reflected by communitywide GPCR Dock 2010 assessment. Structure. 2011;19:1108-26