GC-MS applications in pharmaceutical analysis
Posted: 19 April 2017 | David Elder (David P. Elder Consultancy) | No comments yet
Gas chromatography (GC) predates the more commonly utilised high performance liquid chromatography (HPLC). However, GC continues to enjoy some important niche applications in modern pharmaceutical analysis, such as the analysis of residual solvents or volatile organic compounds (VOCs)…
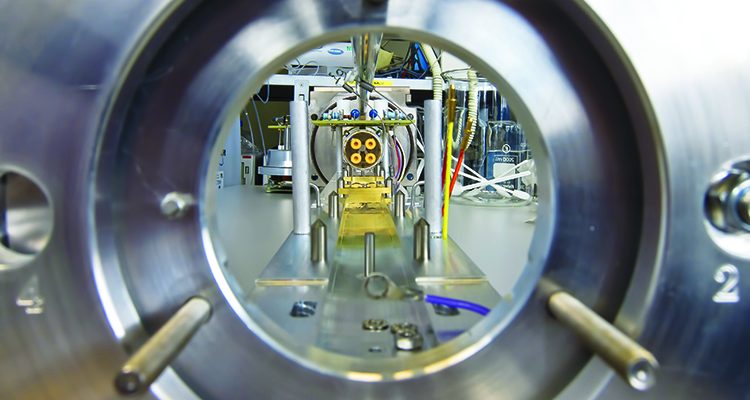
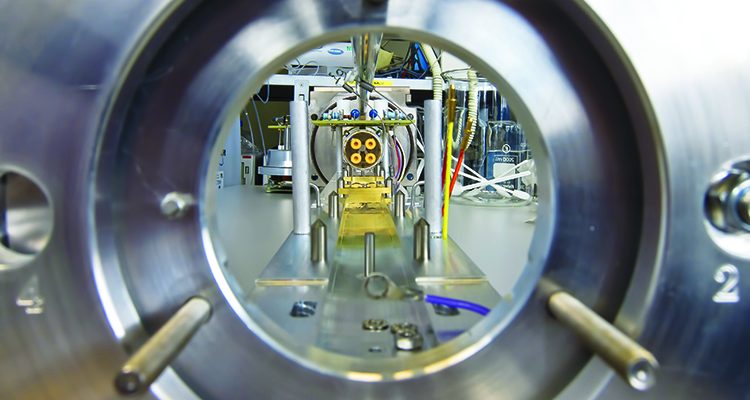
The last decade has seen a renascence of GC – principally in its hyphenated format, ie, GC-MS – where it is now commonly used for the determination of very low levels of volatile mutagenic impurities (MIs)2 such as alkyl halides,3 and in the determination of volatile leachables. This article examines the use of GC and GC-MS in these key areas.
Based on the safety based limits outlined in ICH Q3C(R5),1 GC with standard detection approaches such as flame ionisation detection (FID) or electron capture detection (ECD) has adequate sensitivity to routinely monitor most residual solvents in APIs. However, MS detection may be required if ICH Q3C(R5)1 class 1 solvents are being used, or if there are issues with method selectivity. Recent attention has been focussed on headspace (HS) or static headspace (SHS) variants because these approaches reduce matrix interference, particularly from the API, thus enhancing sensitivity and selectivity. Additionally, derivatisation can significantly enhance sensitivity.4
The use of high boiling point liquids, such as N-methyl pyrrolidone (NMP), dimethylsulfoxide (DMSO) and dimethylformamide (DMF), can improve selectivity and sensitivity, whereas the use of ionic liquids can increase the activity coefficient of the volatile analyte.5 Other strategies for enhancing selectivity and sensitivity were described by Snow and Bullock.6 Cheng et al described the development and validation of a generic static HS-GC-FID method for the determination of class 2 and 3 solvents7 – 44 analytes in total – in four drug substances. Validation was performed in accordance with ICH Q2(R1) guidelines.8 Specificity evaluations showed that baseline separation between the analytes and diluent (DMSO) were attainable for 75% (33/44) of the solvents tested.
The authors demonstrated that APIs containing more than five different residual solvents, which are present at or around the detection limit (DL), were rare. This was ascribed to a combination of optimising of the API crystallisation, isolation and drying stages (as per ICH Q8(R2)).9 Cheng et al also indicated that for the four selected APIs there were between two and four solvents present at, or near to, their quantification limits (QLs).7 Therefore, the authors indicated that the method specificity was appropriate. However, in those cases where more than five residual solvents are present, the method’s selectivity could be optimised using MS and single ion monitoring (SIM).
Linearity was evaluated and found to be acceptable (0.9990-1.0000). The method bias was ≤2.7% of the true values. The repeatability and intermediate precision data were very similar and fit for the intended purpose. The DLs ranged from 0.02-7.41ppm, whereas the QLs ranged from 0.07-24.70ppm. Solvents containing chlorine, oxygen or nitrogen have a lower combustion capacity than hydrocarbons by GC-FID methods, leading to reduced sensitivity and correspondingly greater DL/QL values. The QLs are all lower than the corresponding ICH Q3C(R5) limits for residual solvents (≥50 ppm). However, it should be remembered that process capability requirements often drive the allowable limits below the existing safety-based ICH Q3C(R5) limits.
Toxic solvents and class 1 solvents are typically avoided for late stage chemistry, but they are still used in upstream chemical reactions. Owing to their increased toxicity and commensurably lower allowable limits, enhanced sensitivity is required for these solvents (benzene, carbon tetrachloride, 1,2-dichloroethane, 1,1-dichloroethane and 1,1,1-trichloroethane), which necessitates GC-MS approaches.
Pávon et al described the development and validation of a programmed temperature vaporiser (PTV)-fast GC-MS-SIM approach for class 1 solvents,10 validated in accordance with the requirements of ICH Q2(R1). Specificity was established by a combination of the chromatography (GC) and the selectivity of MS-SIM detection. 1,1-dichloroethane was base-line resolved from the other four analytes (Rt 3.69 minutes). However, 1,2-dichloroethane and 1,1,1-trichloroethane (Rts 5.69 and 5.73 minutes, respectively), and carbon tetrachloride and benzene (Rts 6.00 and 6.06 minutes, respectively), were not resolved. SIM in the extracted ion chromatogram was therefore utilised. In all cases the analytes demonstrated good linearity and the intercepts of the slopes included zero in all cases.
Repeatability was assessed on five replicates with S/N values of three, and was found to be satisfactory (≤12% RSD). The DLs were in the range 4.9-7.9 ppt, while the QLs were evaluated using a S/N value of 10, and were in the range 15-24 ppt. The validation data showed that the method was fit for purpose and had the necessary sensitivity to routinely analyse class 1 solvents, ie, 2-8 ppm limits (see ICH Q3C(R5)). This method compares favourably with other reported GC methods. Fliszar et al used the less sensitive HS-GC-FID approach for the determination of the class 1 solvent benzene and obtained a DL of 100 ppb, with an acceptable level of precision (2.7% RSD).11 Pavón et al utilised HS-GC-MS, but without the PTV adaptor, and consequently showed slightly higher DLs of eight and 42 ppb for benzene and carbon tetrachloride respectively, but with similar precision levels (7.2 and 13% respectively).12
Volatile mutagenic impurities
Liu et al compared the sensitivity of a GC-MS and a GC-ECD method for the determination of ethyl chloride.14 Whereas the analyte could not be detected at the required sensitivity by GC-ECD, this was easily achievable by GC-MS using SIM at m/z 64.
Several groups have provided guidance for method selection/development strategies for MIs.15,16 In all cases, the first decision gate is method choice and in the majority of cases this is predicated on analyte volatility. GC-MS is the method of choice for volatile MIs, and HPLC-MS is the preferred approach for non-volatile impurities.
Headspace analysis
If the analyte exhibits sufficient vapour pressure to be present in a headspace, then matrix interference can be minimised by dissolving the analyte in a non-volatile solvent. The dissolved sample can be placed in a headspace analyser and a temperature ramping programme developed/optimised to separate the analyte from the matrix. Several different approaches are commonly used – HS, SHS, dynamic headspace (DHS) and headspace-solid phase microextraction (HS-SPME). Typically, sensitivity increases in the general order: SHS < SPME < DHS.15
Derivatisation
These headspace approaches can also be used for those MIs that do not have the intrinsic volatility for GC-MS, but can be derivatised to form a volatile derivative. This approach was applied to the analysis of sulfonic acid esters (SAEs), a class of MIs that attract considerable regulatory focus. Alzaga et al reported on the in situ derivatisation of SAEs using pentafluorothiophenol in water/DMSO.17 This was coupled with an HS-GC-MS method that demonstrated low matrix dependency, good sensitivity (DL 0.11ppm), precision (2.8-10% RSD at 1 ppm) and robustness using deuterated internal standards.
In addition, derivatisation can be employed where the MI is reactive by design, but too reactive for routine analysis. In such cases, derivatisation can both stabilise the analyte and enhance its sensitivity for subsequent MS detection. For example, epoxides are unstable, hydrolysing to the corresponding diol, but they can be stabilised using dimethylamine.14 However, care must be taken as the derivatising agents can also be non-selective. For example, both ethyl sulfonic acid and ethyl chloride can give the same derivative using nucleophilic derivatisation agents.4
Direct analysis
A two-dimensional capillary GC method was developed for the determination of various MIs using direct analysis.18 The first-dimension separation used an apolar stationary phase and the fraction (or heart-cut) containing the MIs was switched to a second polar capillary column, which used a low-thermal-mass oven (LTM). The LTM focusses the MI heart-cut(s) and facilitates separate independent temperature-programmed analysis with the second stationary phase. MS contamination and column deterioration are avoided, as the API, solvent and derivatisation agents are not introduced onto the second column. In addition, this improves analyte peak detection and quantification issues. The method was applied to the analysis of various halo-alcohols and Michael acceptor MIs in carbamazepine API. Low detection limits (<1 ppm) and good reproducibility (<10% RSD) at the QLs were obtained.18
Process analytical technology applications
PAT applications and real-time monitoring of MIs are an interesting application. The product quality research institute (PQRI) sub-group assessed the probability of formation of ethyl methane sulfonic acid (EMS) from ethanol and methanesulfonic acid under various reaction conditions. They used automated sampling, derivatisation and reaction quenching allied with on-line SHS-GC-MS methodology to monitor the reaction kinetics of the esterification reaction.19 Similar studies were performed to monitor the purging of methyl iodine during the synthesis of ephedrine using GC-MS.15
Extractables and leachables
Extractables and leachables (E&Ls) studies using GC-MS are designed to analyse volatile and semi-volatile (via derivatisation) compounds from medical devices and container closure systems used for liquid products.20 In some cases GC-MS is used in tandem with HPLC-MS to confirm the identities of unknown E&Ls.21 Armstrong et al highlighted the use of stir bar sorptive extraction (SBSE) combined with GC-MS/MS and multiple reaction monitoring (MRM) for the determination of low-level (150ng/device, ie, < 0.15µg/day) leachable components from implantable medical devices. 22
Future directions
Recent innovations indicate that direct MS applications to quantify both residual solvents and MIs could be feasible, ie, no chromatography will be required. Selected ion flow tube (SIFT)-MS is a direct technique for real-time, comprehensive headspace analysis to very low levels with a wide dynamic range. SIFT-MS couples ultrasoft, precisely controlled CI with MS detection to rapidly quantify VOCs.23 Eight CI reagent ions are utilised in SIFT-MS instruments: H3O+, NO+, O2+, O–, O2–, OH–, NO2– and NO3–. These reagent ions react with VOCs in controlled ion-molecule reactions. This enables SIFT-MS to analyse headspace gases at trace and ultratrace levels without pre-concentration. Rapid switching of the eight reagent ions provides higher selectivity than other direct mass spectrometry techniques. A recent comparative study found a high correlation between SIFT-MS and GC-MS.
Devenport et al reported on the direct detection of a sulfonate ester MI by atmospheric pressure thermal desorption-extractive electrospray (APTD-EE)-MS.25 The analyte is desorbed and vaporised from the matrix by rapid heating and cooling from 200°C, with a cycle time of six minutes. The DL is an order of magnitude below the default threshold of toxicological concern (TTC) of 1.5µg/day.
Conclusions
GC and GC-MS are routinely used for the analysis of volatile impurities in APIs. In those cases where the prevailing safety-based limits are quite high – ie, 5,000 ppm for class 3 solvents – then it makes sense to use the less sophisticated, more robust GDC-FID or GC-ECD approaches. In contrast, where the safety-based limits are low – ie, class 1 solvents, MIs, or volatile leachables – there is a requirement to use the more sensitive GC-MS, often with sample pre-treatment or pre-concentration, ie, HS-GC-MS. If MS is used in the SIM, SRM or MRM modes, additional selectivity and sensitivity are attainable. Future MS-alone developments could revolutionise residual solvent, leachable and MI analysis.
Biography
References
- ICH Q3C(5), 2011. Impurities: Guidelines for residual solvents. Current step 4 version, 04 February 2011.
- ICH M7, 2014. Assessment and control of DNA reactive (mutagenic) impurities in pharmaceuticals to limit potential carcinogenic risk. Current step 4 version, 23 June 2014.
- Elder DP, Teasdale A, et al. The control and analysis of alkyl esters of alkyl and benzyl halides and other related reactive organohalides as potential genotoxic impurities in active pharmaceutical ingredients (APIs). J Pharm Biomed Anal. 2008;48:497-507.
- Orata F. 2012. Derivatization Reactions and Reagents for Gas Chromatography Analysis. http://cdn.intechopen.com/pdfs/32817.pdf. Accessed on 18 March 2017.
- Laus G, Andre M, et al. Ionic liquids as superior solvents for headspace gas chromatography of residual solvents with very low vapour pressure, relevant for pharmaceutical final dosage form. J Chromatogr A. 2009;1216:6020-6023.
- Snow NH, Bullock GP. Novel techniques for enhancing sensitivity in static headspace extraction-gas chromatography. Chromatogr A. 2010;1217:2726-2735.
- Cheng C, Liu S, et al. A generic static headspace gas chromatography method for determination of residual solvents in drug substance. J Chromatogr A. 2010;1217:6413-6421.
- ICH Q2(R1). Validation of analytical procedures: Text and methodology. Current step 4 version, November 2005.
- ICH Q8(R2). Pharmaceutical Development. Current step 4 version, August 2009.
- Pavón JLP, Sánchez MdN, et al. Analysis of class 1 residual solvents in pharmaceuticals using headspace programmed temperature vaporisation-mass spectrometry. J. Chromatogr. A. 2007;1141:123-130.
- Fliszar K, Wiggins JM, et al. Analysis of organic volatile impurities in pharmaceutical excipients by static headspace capillary gas chromatography. J Chromatogr A. 2004;1027:83-91.
- Pavón JLP, Sánchez MdN, et al. Use of mass spectrometry methods as a strategy for detection and determination of residual solvents in pharmaceutical products. Anal Chem. 2006;78:4901-4908.
- Sun M, Liu DQ, Kord AS. A systematic method development strategy for the determination of pharmaceutical genotoxic impurities. Org Proc Res Dev. 2010;14:977-985.
- Liu DQ, Sun M, Korda AS. Recent advances in trace analysis of pharmaceutical genotoxic impurities. J Pharm Biomed Anal. 2010;51(5):999-1014.
- David F, Alegre MR, Vanhoenacker G, Sandra P. Determination of genotoxic impurities in pharmaceuticals. LCGC. May 2013:31-34. http://www.chromatographyonline.com/determination-genotoxic-impurities-pharmaceuticals. Accessed on 18 March 2017.
- Pierson DA, Olsen BA, Robbins DK, DeVries KM, Varie DL. Approaches to assessment, testing decisions, and analytical determination of genotoxic impurities in drug substances. Org Proc Res Dev. 2009;13(2):285-291.
- Alzaga R, Ryan RW, Taylor-Worth K, Lipczynski AM, Szucs R, Sandra P. A generic approach for the determination of residues of alkylating agents in active pharmaceutical ingredients by in situ derivatization-headspace-gas chromatography-mass spectrometry. J Pharm Biomed Anal 2007;45(3):472-479.
- David F, Jacq K, Sandra P, Baker A, Klee MS. Analysis of potential genotoxic impurities in pharmaceuticals by two-dimensional gas chromatography with Deans switching and independent column temperature control using a low-thermal-mass oven module. Anal Biochem Anal. 2010;396(3):1291-1300.
- Jacq K, Delaney E, Teasdale, A, Eyley S, Taylor-Worth K, Lipczynski AM, et al. Development and validation of an automated static headspace gas chromatography-mass spectrometry (SHS-GC-MS) method for monitoring the formation of ethyl methane sulfonate from ethanol and methane sulfonic acid. J Pharm Biomed Anal. 2008;48(5):1339-1344.
- Wong DM, Firor RL. Analysis of Extractable/Leachable Compounds from Generic Liquid Drug Formulations Using GC/MSD Systems. 2015. http://www.agilent.com/cs/library/applications/5991-5632EN.pdf. Accessed on 18 March 2017.
- Pan C, Harmon F, Toscano K, Liu F, Vivilecchia R. Strategy for identification of leachables in packaged pharmaceutical liquid formulations. J Pharm Sci. 2008;46:520-527.
- Armstrong BL, Senyurt AF, Narayan V, Wang X, Alquier L, Vas G. Stir bar sorptive extraction combined with GC-MS/MS for determination of low level leachable components from implantable medical devices. J Pharm Biomed Anal. 2013;74:162-170.
- Perkins MJ, Langford VS, McEwan MJ. High-Throughput VOC and Inorganic Gas Analysis: Automated SIFT-MS. 2017. http://www.labcompare.com/10-Featured-Articles/332740-High-Throughput-VOC-and-Inorganic-Gas-Analysis-Automated-SIFT-MS/?catid=1352. Accessed on 18 March 2017.
- Langford VS, Graves I, et al. Rapid monitoring of volatile organic compounds: A comparison between gas chromatography/mass spectrometry and selected ion flow tube mass spectrometry. Rapid Commun Mass Spectrom. 2014;28:10.
- Devenport NA, Sealey LC, Alruways FH, Weston DJ, Reynolds JC, Creaser CS. Direct detection of sulfonate ester genotoxic impurity by atmospheric pressure thermal desorption-extractive electrospray-mass spectrometry. Anal Chem. 2013;85(13):6224-6227.