NMR spectroscopy: a superior tool for quality control of pharmaceutical products
Posted: 28 October 2014 | | No comments yet
Pharmaceutical products have to be of high quality with respect to a number of predefined attributes, e.g. identity and strength with respect to the active pharmaceutical ingredient (API) and the presence and levels of impurities. At least for smaller compounds, such quality control often involves chromatographic methods. The examples given in this work show that Nuclear Magnetic Resonance (NMR) spectroscopy provides similar but even more detailed quality-related information.
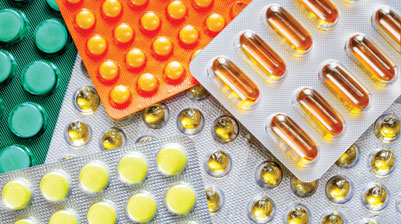
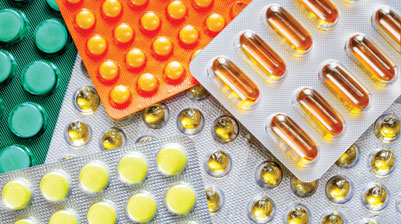
Liquids NMR spectroscopy is well suited for determining the identity of most organic substances, even for such cases where reference substances/spectra are not available1. Due to equal response for any dissolved compound, NMR spectroscopy is also an excellent technique for quantification of any substance in the sample2. Examples include determination of the purity of APIs as well as quantification of active ingredients, excipients and impurities in pharmaceutical products3. Besides this, numerous other physical-chemical properties can be studied, e.g. ligand-receptor binding, diffusion properties, and three-dimensional structure.
The sensitivity as well as the resolution of an NMR instrument increases with the magnetic field strength of the supra-conducting magnet. The strength is normally defined as the proton resonance frequency, today ranging from 300 to over 1000MHz. Since Fourier-transform instruments are used, the longer the measurement time the higher is the signal-tonoise ratio of the analyte spectrum. For dilute samples and proteins, measurements may run for a few days if needed. Besides using a high-field instrument, a cryogenically cooled probehead may further increase the sensitivity 3-4 times, thereby enabling sample amounts lower than 1 microgram for proton spectroscopy. It should be noted that sensitivity varies a lot between different nuclei, depending on the resonance frequency of the particular nucleus (isotope) as well as its natural abundance (Table 1, page 51).
Each type of NMR active nucleus in a molecule results in a specific signal in the NMR spectrum. Its resonance frequency is reported as the chemical shift (ppm) with respect to instrument independent calibrant compounds. Tetramethylsilane is the common chemical shift standard for proton and carbon spectra.
Identification can be performed by comparison to a proper reference standard or reference spectrum of the analyte. The two spectra should preferably be acquired using identical temperature as well as solvent. Due to the occurrence of a phenomenon called spin-spin coupling, proton spectra, and sometimes fluorine and phosphorous spectra, may look different at different magnetic field strengths, and it is therefore recommended that spectra are acquired on instruments having the same field strength. On the other hand, carbon-13 and HSQC spectra are relatively field-independent, i.e. they look reasonably similar at different fields. In such cases spectra from different spectrometers are easily comparable. Searches towards spectra databases are very useful when the structure of the analyte is unknown, particularly for Heteronuclear Single Quantum Coherence (HSQC) and carbon spectra.
When no reference spectrum is available, identification has to be performed by spectral interpretation[i]. Various types of experiments may be used, providing different structural information (Table 2). The achieved spectral data is interpreted either by confirmation/exclusion of one or more target structures or by ‘puzzling’ structure fragments together to a proposed chemical structure that subsequently might be confirmed (spectral interpretation).
Sample preparation is quite simple; usually a few milligrams of a pure compound, or alternatively some tens of milligrams of a pharmaceutical product, is mixed with about 1ml of a deuterated solvent. The solution is transferred to an NMR tube. Undissolved material does not normally cause much disturbance to the measurement, and filtration is not frequently required. For quantitative measurements there are two different approaches[ii]: 1) the internal standard approach, where an exact amount of a suitable internal reference substance is co-dissolved with the sample, and 2) the external standard approach, where the reference and sample solutions are measured in separate NMR tubes.
Validation and structure determination
Pharmaceuticals are often prepared as tablets or capsules. NMR spectra of such products provide very detailed identity information with respect to the API as well as excipients. If a reference spectrum is available, most often a simple proton NMR spectrum is sufficient to confirm identity. Such a case is shown in Figure 1, where part of the 600MHz proton spectrum of a commercial simvastatin tablet is compared to reference spectra of the API, simvastatin, and the major excipient, lactose. Smaller peaks from other excipients are also visible in the spectrum, e.g. ascorbic acid at 3.7 and 3.9ppm.
Many NMR active nuclei (see Table 1) and/or experiment types may be used to confirm the identity of a sample. For one-dimensional carbon, fluorine, phosphorous, and nitrogen spectra the chemical shift range covers hundreds of ppm, resulting in excellent resolution with respect to the magnetic environment around the respective nucleus. The NMR spectrum is a detailed fingerprint that displays what types of functional groups that are present as well as their position in the chemical structure (‘functional groups’).
Much improved spectral dispersion can be achieved by correlation spectroscopy such as Correlated Spectroscopy (COSY), HSQC, and many other experiments. In such experiments, the NMR data is distributed in two or more dimensions, resulting in much less spectral overlap (‘improved resolution’). Drawbacks are increased measurement time and decreased sensitivity. Heteronuclear two-dimensional spectra of small and medium-sized molecules can often be achieved within less time than an hour, while experiments on natural isotopic abundance proteins may require measurement times of one day or more. The example shown in Figure 2 for the simvastatin tablet illustrates the excellent spectral dispersion of all peaks in the proton-carbon HSQC spectrum. The lactose peaks that show severe overlap in the 3.4 to 4ppm region in the proton spectrum (Figure 1) are fully resolved in the HSQC spectrum. The identity of the API as well as the excipients is easily confirmed by comparison to reference spectra of the respective pure compounds.
Another typical example of a correlation spectrum is shown in Figure 3 for the 10 amino acids pharmaceutical peptide nafarelin acetate. About 16mg was dissolved in 1ml water, using a 1 hour measurement time for the proton-nitrogen correlated HSQC experiment on a 600MHz instrument equipped with a cryogenically chilled probe. Amide nitrogen signals were detected in the spectrum, resulting in one unique peak for each amino acid in the peptide back-bone. In some cases peaks from side-chains occur in the same spectral region, as with, for example, tryptophan. A very detailed fingerprint of the peptide structure is obtained, where small changes to the primary and/or the three-dimensional structure would result in a distinct but specific change in the spectrum. Furthermore, if one assigns each peak to each respective amino acid (‘spectral interpretation’), any structural change can be tracked back to a particular part (amino acid) of the peptide chain. It is clear that the possibility to study/follow structural changes is particularly useful for the quality control of macromolecules, such as proteins, since even small changes in the primary or higher order structure may result in remarkably altered pharmacological properties.
Quantification
Proton NMR spectroscopy is probably the most universal technique for quantification of organic compounds. The performance is similar to titration as well as high-performance and gas chromatography. There is no need for a specific reference compound of the analyte, which means that the purity of any compound can be determined by the use of just one or a few reference compounds. In the internal standard approach, the analyte and reference are measured simultaneously, both having equal response. Numerous instrumental and sample errors that may occur in the external standard approach (when sample and reference are measured separately) are eliminated, and time-consuming calibration of the equipment is almost unnecessary. A very useful application of qNMR is determination of the content of API in a pharmaceutical preparation (tablets etc.), both for licensed as well as for various kinds of illegal medicines. One example is described below, illustrating the quantification of a corticosteroid in an ointment.
Creams, ointments and similar products are often regarded as difficult to analyse due to the presence of various fats and emulsions in the formulation. In the selected sample preparation method, a solvent mixture of deuterated chloroform and deuterated methanol was used in order to dissolve as much as possible of all compounds in the product. Thereby, maximum quantitative recovery of both the analyte and the internal standard in the final NMR sample could be expected, provided that the weighed amount of cream or ointment was less than 100mg. This method was successfully applied to various corticosteroid products6 as well as to prohibited hydroquinone in skin-bleaching cosmetic products. The spectrum of a 1% hydrocortisone ointment, shown in Figure 4, displays huge peaks from fatty acids, water and other excipients between 0 and 4.5ppm. Fortunately, the spectral regions at higher ppm are often free from NMR signals, and the analyte peak at 5.6ppm and standard peak at 8.4ppm could easily be integrated. The content of hydrocortisone was calculated to 0.97%, in agreement with the specified content, with an uncertainty of ±0.2% for three sample preparations. This example clearly shows the potential of proton NMR for quantitative purposes, even for analysis of complex sample matrixes. In principle, any NMR active nucleus can be used for quantification, but neither carbon, fluorine nor phosphorous NMR can compete with the sensitivity, accuracy and precision of quantitative proton NMR.
Concluding remarks
To summarise, NMR spectroscopy has proven to be very useful for structure confirmation and elucidation of small organic compounds as well as biomacromolecules. NMR is also a true quantitative technique, with equal response for any compound in a mixture. Recent developments in cryogenically chilled electronics, miniaturisation and stronger magnets have much improved the sensitivity, and NMR can today be applied to most analytical problems.
References
- T.D.W. Claridge, High-Resolution NMR Techniques in Organic Chemistry, Tetrahedron Organic Chemistry Series, Volume 19, Elsevier, Amsterdam, 1999.
- D.L. Rabenstein, D.A. Keire, Quantitative Chemical Analysis by NMR, Practical Spectroscopy, 1991, 11, 323-369.
- U. Holzgrabe, I. Wawer, B. Diehl, NMR Spectroscopy in Pharmaceutical Analysis, Elsevier, Amsterdam, 2008.
- E. Breitmaier, Structure Elucidation by NMR in Organic Chemistry – A Practical Guide, John Wiley & Sons, Chichester, 1993
- T. Rundlöf, Ian McEwen, M. Johansson, T. Arvidsson, Use and Qualification of Primary and Secondary Standards Employed in Quantitative 1H NMR Spectroscopy of Pharmaceuticals, J. Pharm. Biomed. Anal., 2014, 93, 111-117.
- I. McEwen, A. Elmsjö, A. Lehnström, B. Hakkarainen, M. Johansson, Screening of Counterfeit Corticosteroid in Creams and Ointments by NMR Spectroscopy, J. Pharm. Biomed. Anal., 2012, 70, 245-250.
Biography
Torgny Rundlöf is a pharmaceutical evaluator at the laboratory of the Swedish Medical Products Agency. He received a M.S. in chemistry in 1993 and a Ph.D. in organic chemistry in 1998 at Stockholm University. The Ph.D. study was carried out with Professor Göran Widmalm and focused on NMR studies of the structure and dynamics of carbohydrates. In 2000 he joined the MPA as an NMR specialist. His recent work deals with supervision of different kinds of illegal as well as registered medicinal products, using NMR spectroscopy as the main technique. He has also developed quantitative NMR methods for application on various types of medicinal substances and products. Much of this work has been published. Currently he examines the potential of NMR spectroscopy for the quality control of biopharmaceuticals.