Novel strategies for antidepressant drug discovery
Posted: 27 March 2007 | | No comments yet
Despite marked advances in our understanding of chemical transmission, many of the complex processes, namely, our appreciation of the varying roles of neurochemicals in disease etiology, are still being investigated. To aid in this exploration and to monitor the extracellular levels of neurochemicals in living tissue systems, techniques such as in vivo microdialysis are routinely used.
Despite marked advances in our understanding of chemical transmission, many of the complex processes, namely, our appreciation of the varying roles of neurochemicals in disease etiology, are still being investigated. To aid in this exploration and to monitor the extracellular levels of neurochemicals in living tissue systems, techniques such as in vivo microdialysis are routinely used.
Despite marked advances in our understanding of chemical transmission, many of the complex processes, namely, our appreciation of the varying roles of neurochemicals in disease etiology, are still being investigated. To aid in this exploration and to monitor the extracellular levels of neurochemicals in living tissue systems, techniques such as in vivo microdialysis are routinely used.
From a preclinical drug discovery perspective, microdialysis is proving to be a valuable tool in the investigation of neuropsychiatric disease pathology, as well as in the identification of potential new therapies to treat such disorders. The scope of this review article will be to summarise the utility of in vivo microdialysis with a specific emphasis on employing this technique for the discovery of novel antidepressants.
In Vivo microdialysis
In vivo microdialysis provides a unique approach to advance our understanding of brain neurochemistry. Furthermore, this technique offers a potential avenue to investigate the etiology of neuropsychiatric disorders, as well as to facilitate the discovery of novel, disease-modifying pharmacotherapies. In its most elementary form, microdialysis provides a ‘snap shot’ of the local environment, revealing important features of this milieu under baseline conditions as well as the response of this system during pharmacological (or physiological) manipulation. Although this technique was originally described in the field of neuroscience, today microdialysis is common in pharmacokinetic, pharmacodynamic and now, more recently, in clinical study design1,2.
Microdialysis techniques have been used extensively since the late 1960s and early 1970s. It is generally accepted that what we know today as in vivo microdialysis stemmed from groundbreaking work performed by Ungerstedt and colleagues3. These early research efforts resulted in the appropriate refinement of this technique and moved toward coupling the microdialysis sample collection process to what was, at the time, cutting edge liquid chromatography and electrochemical techniques. This union of in vivo sampling with analytical chemistry rapidly expanded the field of microdialysis, ultimately leading to the commercialisation of the technique. In the 21st century, in vivo microdialysis is routinely performed in a variety of freely moving, unanaesthetised animals including mice, rats, guinea pigs, monkeys and humans. Moreover, microdialysis systems can be fully automated (or on-line) in terms of the collection and analysis of dialysis samples and today the expertise of microdialysis has quickly become a common fixture in the pharmaceutical industry. The remaining sections of this review will summarise how in vivo microdialysis is facilitating the discovery of novel antidepressants.
The monoamine hypothesis of depression
It is postulated that the debilitating and often chronic symptoms of depression result from a perturbation of monoamine neurochemistry. This hypothesis, in large part, spawned from early work showing that monoamine oxidase inhibitors and tricyclics, which elevate the levels of monoamines by preventing their breakdown and reuptake, respectively, were effective antidepressant agents4. In the late 1980s, these approaches to treat depression were surpassed by the selective serotonin reuptake inhibitors (SSRIs), which work to increase central levels of serotonin (or 5-hydroxytryptamine; 5-HT). The most recent class of effective antidepressants are the dual serotonin / noradrenaline reuptake inhibitors (SNRIs). By comparison to other classes, SNRIs such as Effexor® are suggested to exhibit a faster onset of antidepressant action5,6, while being more effective in treating refractory depression7,8. Overall, these results support the monoamine hypothesis of depression as well as highlight the importance of elevating levels of neurotransmitters such as 5-HT and noradrenaline (NA) in the clinical management of depression. Despite the success of these classes of antidepressants, there continues to be considerable unmet medical needs both in terms of efficacy and side effects9. Thus, there is a clear need for innovative strategies to either further ‘tweak’ the monoamine system and / or target other neurochemical (e.g., neuropeptide) systems. The following sections will highlight how in vivo microdialysis has been useful in facilitating the discovery of such innovative antidepressant strategies.
Microdialysis and novel strategies to discover antidepressants
Broad-spectrum antidepressants: Triple monoamine uptake inhibition
While the monoamine hypothesis of depression primarily focuses on 5-HT and NA, considerable reports suggest an emerging role for dopamine (DA) in the core symptoms of depression (e.g., anhedonia)10. Given this evidence, coupled to the clinical success of SSRIs and SNRIs, a plethora of information suggests that elevating extracellular levels of all three monoamines may be advantageous for the treatment of depression. This ‘broad-spectrum’ approach is gaining momentum largely due to the recent description of DOV 21,947, a single molecule possessing potent inhibition of the reuptake of NA, 5-HT, and DA11. In rodents, DOV 21,947 produces antidepressant-like activity in the forced swim and tail suspension tests11. Additionally, our research group has profiled this compound using in vivo microdialysis. Consistent with its in vitro potency to block the uptake of all three monoamines, DOV 21,947 elevated the extracellular levels of NA, 5-HT, and DA in the rat medial prefrontal cortex (Figure 1). This neurochemical strategy for antidepressant development is particularly amenable for evaluation by microdialysis and has yielded preclinical data that nicely complements the effects of DOV 21,947 in behavioural models of depression11. However, despite these early reports and preclinical validation of mechanism, the overall benefit of inhibiting all three monoamines via a single molecule is still awaiting rigorous clinical valuation.
Combination strategies: SSRIs plus autoreceptor antagonists
Even in the approximate 50 per cent of patients that do respond to therapy, a common characteristic of all marketed antidepressants, regardless of their mechanism of action, is the 4 to 6 week delay required to reach clinical efficacy. The prominent hypothesis surrounding this delay implies that desensitisation of 5-HT1A autoreceptors must occur prior to the onset of antidepressant activity. This theory is supported by in vivo microdialysis studies showing that central 5-HT levels are increased following chronic12, but not acute13, treatment with SSRIs. Additional neurochemical data demonstrate that selective 5-HT1A antagonism augments SSRI- and SNRI-induced changes in cortical serotonin levels13. In behavioural models, 5-HT1A receptor antagonists potentiate the antidepressant-like effects of SSRIs in the rodent resident-intruder, social interaction and schedule-induced polydipsia assays14,15,16. These collective preclinical results, as well as clinical data combining antidepressants with the mixed b-adrenergic / 5-HT1A ligand, pindolol17, strongly suggest that a strategy to combine SSRIs with 5-HT1A receptor antagonism should yield an effective antidepressant that elicits rapid and robust increases in 5-HT. Correspondingly, several companies have recently published the synthesis of such ‘dual-acting’ compounds possessing potent activity as both SSRIs and full / partial 5-HT1A receptor antagonists. One example of such molecules is WAY-211612, which, as revealed by in vivo microdialysis, acutely elevates cortical 5-HT levels as well as exhibits antidepressant-like activity in rodent models of depression (i.e., resident intruder) and anxiety (i.e., 4-plate and schedule-induced polydipsia)18. Despite these promising results, it remains to be seen whether this combination approach will represent the next generation of novel and ‘fast-acting’ antidepressants.
In addition to 5-HT1A receptors, chronic treatment with several classes of antidepressants, particularly NA reuptake inhibitors (NRIs) and the SNRIs, are reported to desensitise a-2 adrenergic autoreceptors. Thus, antidepressant-induced release of NA activates α-2 adrenergic autoreceptors located on noradrenergic neurons resulting in a blunted neurochemical response. Furthermore, since 5-HT efferents from the dorsal raphe are inhibited by local α-2 adrenergic receptors, blocking α-2 receptors may also influence serotonergic output19. Based on this rationale, it is postulated that antidepressants, when given in combination with agents that ‘turn off’ α-2 autoreceptors, should acutely increase levels of both NA and 5-HT. In vivo microdialysis experiments corroborate this approach and illustrate that non-selective α-2 and selective α-2A adrenergic antagonists, such as idazoxan and BRL-44408, respectively, markedly potentiate the ability of antidepressants to increase extracellular levels of NA and 5-HT acutely19,20. While there is limited published data showing that this particular combination strategy is superior to the effects of marketed antidepressants in behavioural models, the data generated from microdialysis experiments suggest that antagonism of α-2 adrenergic receptors may improve the neurochemical effects of antidepressants and, consequently, may improve the efficacy of antidepressants in humans. Some clinical studies support this concept and show that combining SSRIs with nonselective α-2 receptor antagonists (e.g., mirtazapine and yohimbine) actually shortens the time required to achieve antidepressant activity21,22. Similar to other approaches, this neurochemical hypothesis has ignited considerable chemistry efforts to design and synthesise novel molecules that combine monoamine reuptake inhibition with α-2 adrenergic receptor antagonism23.
Subtype selective antidepressants: 5-HT6 receptor agonists
Our research group has recently employed in vivo microdialysis to characterise the novel and selective 5-HT6 receptor agonists, WAY-181187 and WAY-20846624. Consistent with the localisation of these receptors on GABAergic cell bodies25, acute 5-HT6 receptor stimulation elicited significant increases in GABA levels in cortico-limbic brain areas such as the frontal cortex, striatum, dorsal hippocampus and amygdala24. Interestingly, these GABAergic effects were greater in magnitude following chronic (14-day) administration of WAY-20846624. Given that disruptions in GABA transmission have been linked to major depressive and anxiety disorders in humans26, these microdialysis results potentially indicate that 5-HT6 receptor stimulation may represent a novel strategy to treat neuropsychiatric disorders. Consistent with this hypothesis, acute administration of 5-HT6 receptor agonists, at doses shown to elevate GABA levels in microdialysis experiments, exhibit antidepressant and anti-obsessive compulsive-like activity in the rat forced swim test27 and schedule-induced polydipsia model24, respectively. Similar to the previous strategies discussed, the use of 5-HT6 receptor agonists in the treatment of major depressive and anxiety disorders is still awaiting clinical examination. What is, however, interesting to note is that all of these new strategies are being interrogated, at least in part, by in vivo microdialysis techniques. In the next several years, as some of these innovative strategies become marketed antidepressants, it will be important to look back retrospectively and re-evaluate the data generated by microdialysis (as well as other models) to appreciate how meaningful this preclinical information is in predicting efficacy in patients.
Beyond monoamines: unleashing the potential of microdialysis
The strategies described above seem to efficiently ‘tweak’ the monoaminergic systems in the hopes of developing more effective and rapid-acting antidepressants. However, considerable clinical data surrounding these various approaches in terms of efficacy and tolerability is eagerly anticipated. It has been proposed that the most novel and innovative strategies to treat depression may indeed be elucidated by targeting convergent, down-stream components of the monoamine system (e.g. neurotrophins) and / or non-monoaminergic systems including GABAergic and glutamatergic circuitries. An example of some – but by no means all – of these novel antidepressant strategies reported in the literature include peptide targets such as the corticotrophin releasing factor (CRF), substance P, melanin concentrating hormone (MCH) and vasopressin28. Similarly, there has been considerable excitement recently regarding antidepressant-induced neurogenesis and the precise role of this phenomenon in the neurobiology of neuropsychiatric disorders28.
In light of data hinting at a role for various trophic factors in the potential treatment of depression28, we have focused our microdialysis studies to better evaluate how such novel targets (e.g. insulin-like growth factor; IGF and brain-derived neurotrophic factor and BDNF) affect brain neurochemistry. A recent example of this research investigated the downstream neurochemical effects of exogenously administered IGF-1. It was discovered that subchronic administration of IGF-1 directly into the brain evoked an approximate two-fold increase in the levels of 5-HT in the hippocampus. While these preliminary findings elucidate a perhaps unobvious influence of IGF-1 on central serotonergic systems, it is neurochemical results such as these that make the technique of microdialysis invaluable to both basic research and pharmaceutical drug discovery. Moreover, without understanding the neurochemical effects of novel treatment strategies, we may not fully appreciate the impact that these targets and our future compounds will have on modifying disease pathology.
Summary
As in vivo microdialysis continues to be coupled to more sophisticated analytical equipment29,30, it is becoming easier to measure molecules that, until this point, have been impossible to monitor with traditional electrochemical or fluorescent detection systems. Furthermore, the combination of this technique with behavioural models allows “real time” information to be simultaneously collected in freely moving animals as they perform various tasks or undergo preclinical facets of disease pathology. In this way, microdialysis serves as a powerful supplement to data generated in classical behavioural models of neuropsychiatric disorders (e.g. forced swim, resident intruder, etc.). Moving toward the future of antidepressant drug discovery, it is clear that techniques such as in vivo microdialysis will play an increasingly important role, not only in compound screening, but also in the preclinical unveiling of novel targets that improve upon efficacy and address the many unmet medical needs in the field of neuropsychiatry.
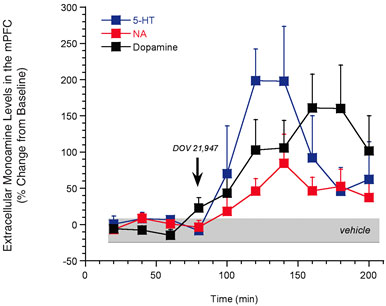
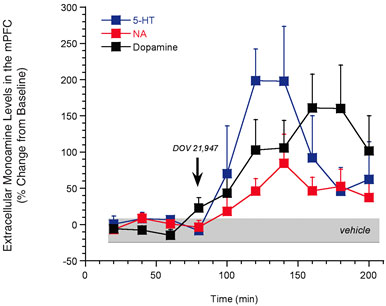
Figure 1: In vivo microdialysis studies demonstrating that acute treatment with DOV 21,947 (10 mg/kg, subcu) significantly increases levels of 5-HT, NA and DA in the rat medial prefrontal cortex (mPFC). Significant (P<0.05) treatment
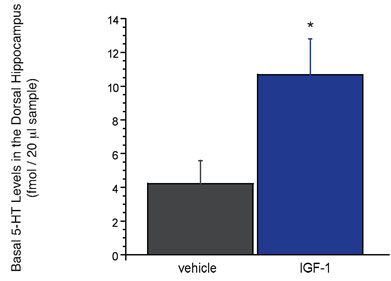
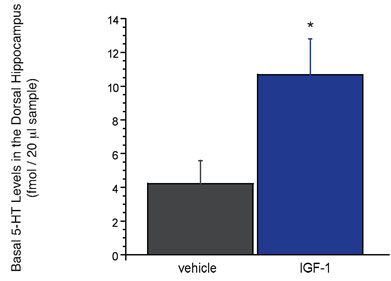
Figure 2: Subchronic (five day) infusion of IGF-1 (1.2 Ìg / per day) into the ventricular system (or intracerebroventricular, icv) increases basal levels of 5-HT in the rat dorsal hippocampus. Effects of IGF-1 that were significantly (P<0.05) different from vehicle are designated with an asterisk
References
- Stahl M, Bouw R, Jackson A, Pay V. 2002. Human microdialysis. Curr Pharm Biotechnol, 3, 165-178
- Joukhadar C, Muller M. 2005. Microdialysis: current applications in clinical pharmacokinetic studies and its potential role in the future. Clin Pharmacokinet. 44, 895-913
- U. Ungerstedt, C. Pycock. 1974. Functional correlates of dopamine neurotransmission. Bull Schweiz Akad Med Wiss. 30, 44-55
- Eriksson E. 2000. Antidepressant drugs: does it matter if they inhibit the reuptake of noradrenaline or serotonin. Acta Psychiatr Scand. 101 (Suppl. 402), 12-17
- Clerc GE, Ruimy P, Verdeay-Pailles J. 1995. A double blind comparison of venlafaxine and fluoxetine in patients hospitalized for major depression and melancholia. Int Clin Psychopharmacol. 9, 138-143
- Guelfi JD, White C, Hackett D, Guichoux JY, Magni G. 1995. Effectiveness of venlafaxine in patients hospitalized for major depression and melancholia. J Clin Psych. 56, 450-458
- de Montigny C, Debonnel G, Bergeron R, St Andre E, Blier P. 1995. Venlafaxine in treatment resistant depression: open label multicentre study. Am Coll Neuropharmacol. 34, 158
- Nierenberg AA, Feigner JP, Rudolph R, Cole JO, Sullivan J. 1994. Venlafaxine for treatment resistant unipolar depression. J Clin Psychopharmacol. 4, 419-423
- Rosenzweig-Lipson S, Beyer CE, Hughes ZA, Khawaja X, Rajarao SJ, Malberg JE, Rahman Z, Ring RH, Schechter LE. 2007. Differentiating antidepressants of the future: Efficacy and safety. Pharmacology & Therapeutics. 113, 134-153
- Randrup A, Braestrup C. 1997. Uptake inhibition of biogenic amines by newer antidepressant drugs: relevance to the dopamine hypothesis of depression. Psychopharmacology. 53, 309-314
- Skolnick P, Popik P, Janowsky A, Beer B, Lippa AS. 2003. Antidepressant-like actions of DOV 21,947: a “triple” reuptake inhibitor. Eur J Pharmacol. 461, 99-104
- Dawson LA, Nguyen HQ, Smith DL, Schechter LE. 2002. Effect of chronic fluoxetine and WAY-100635 treatment on serotonergic neurotransmission in the frontal cortex. J Psychopharmacol. 16, 145-152
- Beyer CE, Boikess S, Luo B, Dawson LA. 2002. Comparison of the effects of antidepressants on norepinephrine and serotonin concentrations in the rat frontal cortex: an in-vivo microdialysis study. J Psychopharmacol. 16, 297-304
- Mitchell PJ, Redfern PH. 1997. Potentiation of the time-dependent, antidepressant-induced changes in the agonistic behaviour of resident rats by the 5-HT1A receptor antagonist, WAY-100635. Behav Pharmacol. 8, 585-606
- Duxon MS, Starr KR, Upton N. 2000. Latency to paroxetine-induced anxiolysis in the rat is reduced by co-administration of the 5-HT(1A) receptor antagonist WAY100635. Br J Pharmacol. 130, 1713-1719
- Hogg S, Dalvi A. 2004. Acceleration of onset of action in schedule-induced polydipsia: combinations of SSRI and 5-HT1A and 5-HT1B receptor antagonists. Pharmacol Biochem Behav. 77, 69-75
- Blier P, Bergeron R. 1998. The use of pindolol to potentiate antidepressant medication. J Clin Psychiatry. 59 (Suppl 5), 16-23
- Beyer CE, Lin Q, Platt B, Malberg JE, Hornby G, Sullivan KM, Smith DL, Hatzenbuhler NT, Evrard DA, Harrison BL, Schechter LE, Rosenzweig-Lipson S, Andree TH. 2006. WAY-211612: neurochemical and behavioral characterization of a dual SSRI / 5-HT1A receptor antagonist and potential novel antidepressant. Society for Neuroscience Conference, Poster, 828.1, Atlanta, GA
- Gobert A, Rivet JM, Cistarelli L, Melon C, Millan MJ. 1997. Alpha2-adrenergic receptor blockade markedly potentiates duloxetine- and fluoxetine-induced increases in noradrenaline, dopamine, and serotonin levels in the frontal cortex of freely moving rats. J Neurochem. 69, 2616-2619
- Beyer CE, Lin Q, Rosenzweig-Lipson S, Schechter LE. 2006. ·2A-adrenoceptors enhance the serotonergic effects of fluoxetine. Eur J Pharmacol. 539, 164-167
- Cappiello A, McDougle CJ, Malison RT, Heninger GR, Price LH. 1995. Yohimbine augmentation of fluvoxamine in refractory depression: a single-blind study. Biol Psychiatry. 38, 765-767
- Sanacora G, Berman RM, Cappiello A, Oren DA, Kugaya A, Liu N, et al. 2004. Addition of the alpha2-antagonist yohimbine to fluoxetine: effects on rate of antidepressant response. Neuropsychopharmacology. 29, 1166-1171
- Andres JI, Alcazar J, Alonso JM, Alvarez RM, Bakker MH, Biesmans I, et al. 2005. Discovery of a new series of centrally active tricyclic isoxazoles combining serotonin (5-HT) reuptake inhibition with alpha2-adrenoceptor blocking activity. J Med Chem. 48, 2054-2071
- Schechter LE, Lin Q, Smith DL, Zhang G, Shan Q, Platt B, Brandt MR, Dawson LA, Cole D, Bernotas R, Robichaud A, Rosenzweig-Lipson S, Beyer CE. 2007. Neuropharmacological Profile of Novel and Selective 5-HT6 Receptor Agonists: WAY-181187 and WAY-208466. Neuropsychopharmacology, Submitted
- Woolley M, Marsden C, Sleight A, Fone K. 2001. Co-localization of 5-HT6 receptors on GABAergic but not cholinergic neurones in the adult brain. Society for Neuroscience Satellite Symposium: Serotonin: From the molecule to the clinic, San Diego, CA
- Sanacora G, Mason GF, Rothman DL, Krystal JH. 2002. Increased occipital cortex GABA concentrations in depressed patients after therapy with selective serotonin reuptake inhibitors. Am J Psych. 159, 663-665
- Schechter LE, Liu D, Bernotas R, Cole D, Robichaud A, Lucki I, Rosenzweig-Lipson S, Platt B, Liang S, Pong K, Zaleska M. 2006. 5-HT6 receptor stimulation regulates BDNF and induces neuronal plasticity: potential mechanisms for antidepressant drug action and neuroprotection. Neuropsychopharmacology. 31(Suppl. 1), S170
- Schechter LE, Ring RH, Beyer CE, Hughes ZA, Khawaja X, Malberg JE, Rosenzweig-Lipson S. 2005. Innovative Approaches for the Development of Antidepressant Drugs: Current and Future Strategies. NeuroRx. 2, 590-611
- Zhang M, Beyer CE. 2006. Measurement of neurotransmitters from extracellular fluid in brain by in vivo microdialysis and chromatography-mass spectrometry. J Pharmaceut Biomedical Analysis. 40, 492-499
- Khandelwal P, Beyer CE, Lin Q, Schechter LE, Bach AC. 2004. Studying rat brain neurochemistry using nanoprobe NMR spectroscopy: a metabonomics approach. Analytical Chem. 76, 4123-4127